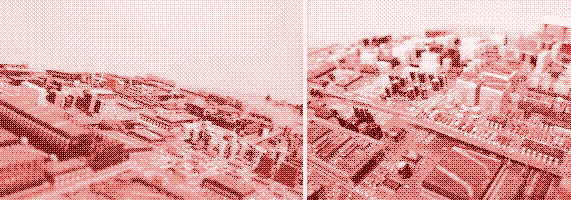
The power consumption of our high-tech machines and devices is hugely underestimated.
When we talk about energy consumption, all attention goes to the electricity use of a device or a machine while in operation. A 30 watt laptop is considered more energy efficient than a 300 watt refrigerator. This may sound logical, but this kind of comparisons does not make much sense if you don’t also consider the energy that was required to manufacture the devices you compare. This is especially true for high-tech products, which are produced by means of extremely material- and energy-intensive manufacturing processes. How much energy do our high-tech gadgets really consume?
The energy consumption of electronic devices is skyrocketing, as was recently reported by the International Energy Association ("Gadgets and gigawatts"). According to the research paper, the electricity consumption of computers, cell phones, flat screen TV’s, iPods and other gadgets will double by 2022 and triple by 2030. This comes down to the need for an additional 280 gigawatts of power generation capacity. An earlier report from the British Energy Saving Trust (The ampere strikes back- pdf) came to similar conclusions.
There are multiple reasons for the growing energy consumption of electronic equipment; more and more people can buy gadgets, more and more gadgets appear, and existing gadgets use more and more energy (in spite of more energy efficient technology - the energy efficiency paradox described here before).
The 180 watt laptop
While these reports are in themselves reason for concern, they hugely underestimate the energy use of electronic equipment. To start with, electricity consumption does not equal energy consumption. In the US, utility stations have an average efficiency of about 35 percent. If a laptop is said to consume 60 watt-hours of electricity, it consumes almost three times as much energy (around 180 watt-hour, or 648 kilojoules).
So, let’s start by multiplying all figures by 3 and we get a more realistic image of the energy consumption of our electronic equipment. Another thing that is too easily forgotten, is the energy use of the infrastructure that supports many technologies; most notably the mobile phone network and the internet (which consists of server farms, routers, switches, optical equipment and the like).
Embodied energy
Most important, however, is the energy required to manufacture all this electronic equipment (both network and, especially, consumer appliances). The energy used to produce electronic gadgets is considerably higher than the energy used during their operation. For most of the 20th century, this was different; manufacturing methods were not so energy-intensive.
An old-fashioned car uses many times more energy during its lifetime (burning gasoline) than during its manufacture. The same goes for a refrigerator or the typical incandescent light bulb: the energy required to manufacture the product pales into insignificance when compared to the energy used during its operation.
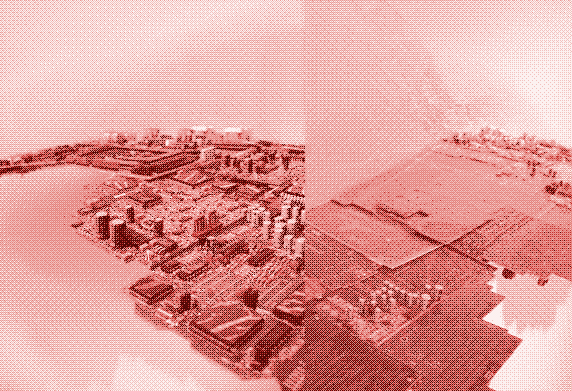
Advanced digital technology has turned this relationship upside down. A handful of microchips can have as much embodied energy as a car. And since digital technology has brought about a plethora of new products, and has also infiltrated almost all existing products, this change has vast consequences. Present-day cars and since long existing analogue devices are now full of microprocessors. Semiconductors (which form the energy-intensive basis of microchips) have also found their applications in ecotech products like solar panels and LEDs.
Where are the figures?
While it is fairly easy to obtain figures regarding the energy consumption of electronic devices during the use phase (you can even measure it yourself using a power meter), it is surprisingly hard to obtain reliable and up-to-date figures on the energy consumed during the production phase. Especially when it concerns fast-evolving technologies. A life cycle analysis of high-tech products is extremely complex and can take many years, due to the large amount of parts, materials and processing techniques involved. In the meantime, products and processing technologies keep evolving, with the result that most life cycle analyses are simply outdated when they are published.
The embodied energy of the memory chip alone already exceeds the energy consumption of a laptop during its life expectancy of 3 years.
For more recent and emerging technologies, life cycle analyses simply do not exist. Try looking for a research paper that calculates the embodied energy of a Light Emitting Diode (LED), a lithium-ion battery or any device full of electronics meant to save energy: you won’t find it (and if you do, please let me know).
Embodied energy of a computer
The most up-to-date life cycle analysis of a computer dates from 2004 and concerns a machine from 1990. It concluded that while the ratio of fossil fuel use to product weight is 2 to 1 for most manufactured products (you need 2 kilograms of fuel for 1 kilogram of product), the ratio is 12 to 1 for a computer (you need 12 kilograms of fuel for 1 kilogram of computer). Considering an average life expectancy of 3 years, this means that the total energy use of a computer is dominated by production (83% or 7,329 megajoule) as opposed to operation (17%). Similar figures were obtained for mobile phones.
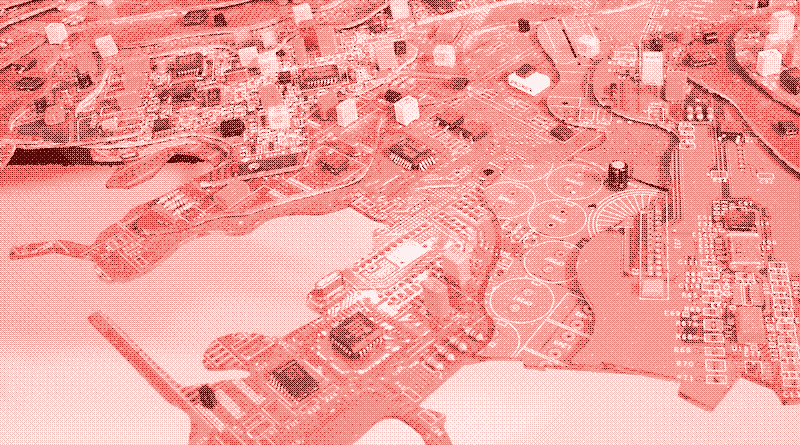
While the 1990 computer was a desktop machine with a CRT-monitor, many of today’s computers are laptops with an LCD-screen. At first sight, this seems to indicate that the embodied energy of today’s machines is lower than that of the 1990 machine, because much less material (plastics, metals, glass) is needed. But it is not the plastic, the metal and the glass that makes computers so energy-instensive to produce. It’s the tiny microchips, and present-day computers have more of them, not less.
100 years of manufacturing
The energy needed to manufacture microchips is disproportional to their size. MIT-researcher Timothy Gutowski compared the material and energy intensity of conventional manufacturing techniques with those used in semiconductor and in nanomaterial production (a technology that is being developed for use in all kinds of products including electronics, solar panels, batteries and LEDs).
Digital technology is a product of cheap energy
As an example of more conventional manufacturing methods, Gutowski calculated the energy requirements of machining, injection molding and casting. All these techniques are still used intensively today, but they were developed almost 100 years ago. Injection molding is used for the manufacture of plastic components, casting is used for the manufacture of metal components, and machining is a material removing process that involves the cutting of metals (used both for creating and finishing products).
6 orders of magnitude
While there are significant differences between configurations, all these manufacturing methods require between 1 and 10 megajoule of electricity per kilogram of material. This corresponds to 278 to 2,780 watt-hour of electricity per kilogram of material. Manufacturing a one kilogram plastic or metal part thus requires as much electricity as operating a flat screen television for 1 to 10 hours (if we assume that the part only undergoes one manufacturing operation).
The energy requirements of semiconductor and nanomaterial manufacturing techniques are much higher than that: up to 6 orders of magnitude (that’s 10 raised to the 6th power) above those of conventional manufacturing processes (see figure below, source, supporting information). This comes down to between 1,000 and 100,000 megajoules per kilogram of material, compared to 1 to 10 megajoules for conventional manufacturing techniques.
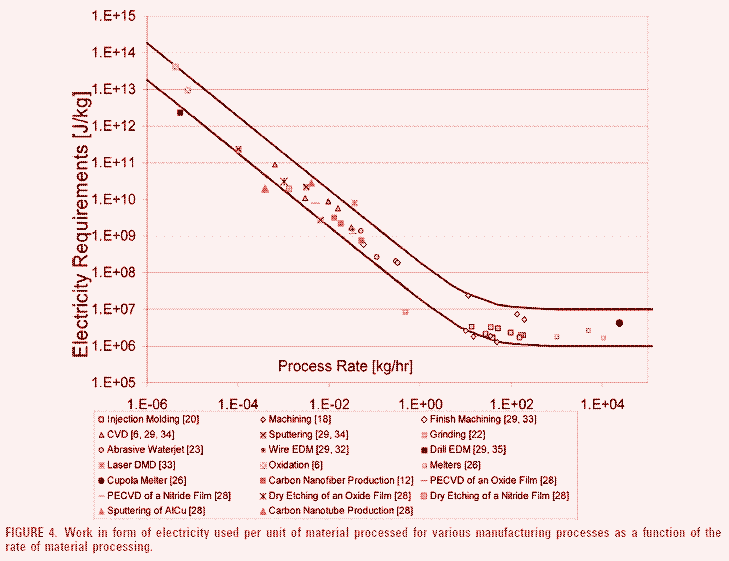
Manufacturing one kilogram of electronics or nanomaterials thus requires between 280 kilowatt-hours and 28 megawatt-hours of electricity; enough to power a flat screen television continuously for 41 days to 114 years. These data do not include facility air handling and environmental conditioning, which for semiconductors can be substantial.
Embodied energy of a microchip
The energy consumption of semiconductor manufacturing techniques corresponds with a life cycle analysis of a “typical” 2 gram microchip performed in 2002. Again, this concerns a 32 MB RAM memory chip - not really cutting edge technology today. But the results are nevertheless significant: to produce the 2 gram microchip, 1.6 kilograms of fuel were needed. That means you need 800 kilograms of fuel to produce one kilogram of microchips, compared to 12 kilograms of fuel to produce one kilogram of computer.
If we take the energy density of crude oil (45 MJ/kg), this comes down to 72 megajoules (or 20,000 watt-hour) to produce a 2 gram microchip. Converted to a one kilogram microchip this comes down to 3.3 megawatt-hours of electricity (or 36,000 MJ), well within the range of the 280 kilowatt-hours (1,000MJ) and 28 megawatt-hours (100,000 MJ) calculated above.
Also, the International Technology Roadmap for Semiconductors 2007 edition gives a figure of 1.9 kilowatt-hours per square centimetre of microchip, so 20 kilowatt-hours per 2 gram, square centimetre computerchip seems to be a reasonable estimate.
How many microchips in a computer?
A gadget or a computer does not contain one kilogram of semiconductors - far from that. But, we don’t need a kilogram of microchips to ensure that the manufacturing phase will largely outweigh the usage phase. The embodied energy of the memory chip alone already exceeds the energy consumption of a laptop during its life expectancy of 3 years.
Today’s personal computers have a RAM-memory of 0.5 to 2 gigabyte modules that typically consist of 18 to 36 two-gram-microchips (as the ones described above). This equates to 1,296 to 2,595 megajoules of embodied energy for the computer memory alone, or 360,000 to 720,000 watt-hour. Enough to power a 30 watt laptop non-stop for 500 to 1,000 days.
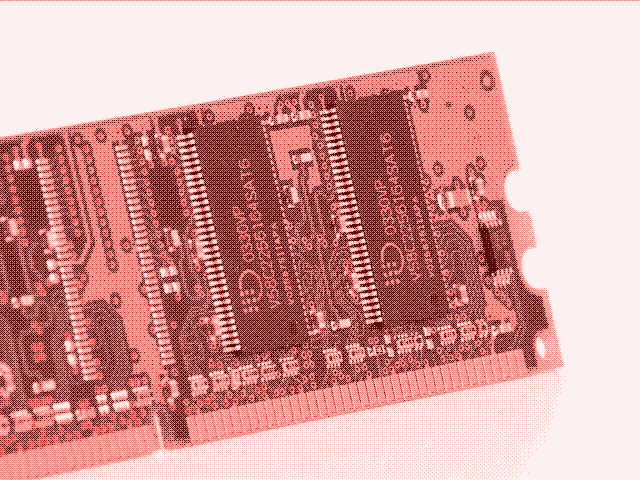
Microprocessors (the “brains” of all digital devices) are more advanced than memory chips and thus contain at least as much embodied energy. Unfortunately, no life cycle analysis of a microprocessor has been published. Certain is that modern computers contain ever more of them.
One trend in recent years is the introduction of “multicore processors” and “multi-CPU systems”. Personal computers can now contain 2, 3 or 4 microprocessors. Servers, game consoles and embedded systems can have many more. Each of these “cores” is capable of handling its own task independently of the others. This makes it possible to run several CPU-intensive processes (like running a virus scan, searching folders or burning a DVD) all at the same time, without a hitch. But with every extra chip (or chip surface) comes more embodied energy.
The energy savings realised by digital technology will merely absorb its own growing footprint.
Another trend is the rise of the “Graphics Processing Unit” or GPU. This is a specialised processor that offloads 3D graphics rendering from the microprocessor. The GPU is indispensable to play modern videogames, but it is also needed because of the ever higher graphical requirements of operating systems. GPU’s do not only raise the energy consumption of a computer while in use (GPU’s can consume more energy than current CPU’s), but they also stand for more embodied energy. A GPU is very memory-intensive and thus also increases the need for more RAM-chips.
Nanomaterials
Why are microchips so energy-intensive to manufacture? One of the reasons becomes clear when you literally zoom in on the technology. A microchip is small, but the amount of detail is fabulous. A microprocessor the size of a fingernail can now contain up to two billion transistors - each transistor less than 0.00007 millimetres wide. Magnify this circuit and it becomes a structure as complex as a sprawling metropolitan city.
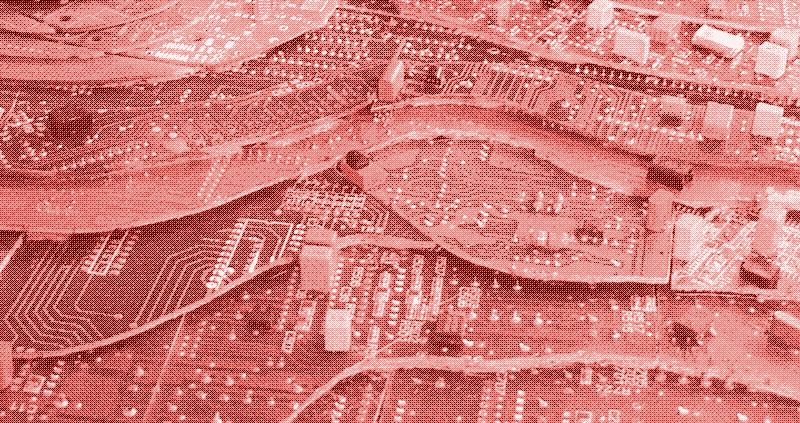
The amount of materials embedded in the product might be small, but it takes a lot of processing (and thus machine energy use) to lay down a complex and detailed circuit like that. While the electricity requirements of machines used for semiconductor manufacturing are similar to those used for older processes like injection molding, the difference lies in the process rate: an injection molding machine can process up to 100 kilograms of material per hour, while semiconductor manufacturing machines only process materials in the order of grams or milligrams.
Another reason why digital technology is so energy-intensive to manufacture is the need for extremely effective air filters and air circulation systems (which is not included in the figures above). When you build infinitesimal structures like that, a speck of dust would destroy the circuit. For the same reason, the manufacture of microchips requires the purest silicon (Electronic Grade Silicon or EGS, provided by the energy-intensive CVD-process).
The manufacture of nanotubes is as energy-intensive as the manufacture of microchips.
Every 18 months the amount of transistors on a microchip doubles (Moore’s law). On one hand, this means that less silicon is needed for a certain amount of processing power or memory. On the other hand, when transistors become smaller, you need even more effective air filtration and purer silicon. Since the structure also becomes more complex, you need more processing steps.
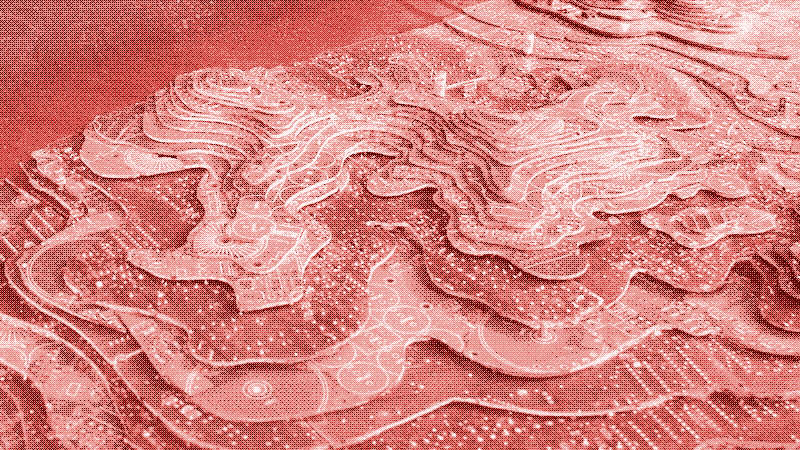
Nanotechnology operates on an even smaller scale than micro-electronics, but its energy requirements are comparable. Carbon nanofiber production, which is based on many of the same techniques used by semiconductor manufacturing, requires 760 to 3,000 MJ of electricity per kilogram of material, while carbon nanotubes and single-walled nanotubes (SWNTs) manufacturing requires a hefty 20,000 to 50,000 MJ per kilogram. The manufacture of nanotubes is thus as energy-intensive as the manufacture of microchips (36,000 MJ). Many of the large-scale applications proposed for nanotubes will simply not be possible because of energy requirements.
Recycling is no solution
Encouraging recycling is often proposed as a way to lower the embodied energy of products. Unfortunately, this does not work for micro-electronics (or nanomaterials). In the case of conventional manufacturing methods, the energy requirements of the manufacturing process (1 to 10 MJ per kilogram) are small compared to the energy required to produce the materials themselves.
For instance, producing 1 kilogram of plastic out of crude oil requires 62 to 108 MJ of energy, while a typical mix of virgin and recycled aluminum requires 219 MJ. To make a fair comparison, you have to multiply the energy requirement of the manufacturing process by three (1 megajoule of electricity requires 3 megajoules of energy) but even then (with 3 to 30 MJ/kg) conventional manufacturing processes appear to be quite benign compared to materials extraction and primary processing (in the order of 100 MJ/kg - see table).
Recycling is not a solution if all your energy use is concentrated in the manufacturing process itself.
In the case of semiconductor manufacturing, this relation is reversed. While it takes 230 to 235 MJ of energy to produce 1 kilogram of silicon (already quite high compared to many other materials), chemical vapour deposition (an important step in the semiconductor manufacturing process) requires about 1,000 MJ of electricity and thus 3,000 MJ of energy per kilogram.
That is 10 times more than the energy consumption of material extraction and primary processing. In the case of conventional manufacturing techniques, the use of recycled material is an effective way to lower overall energy use during manufacture. In the case of semiconductors, it is not. Recycling is not a solution for energy consumption if all your energy use is concentrated in the process itself.
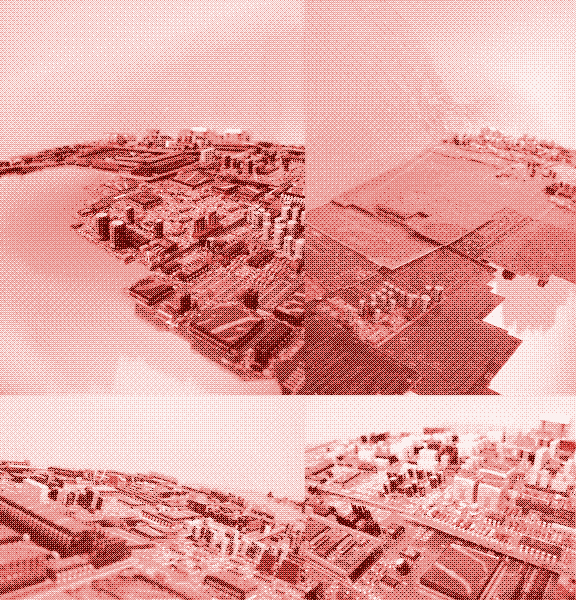
This does not mean that the manufacture of microchips does not require materials. In fact, producing microchips and nanomaterials is also more intensive than the manufacture of conventional products, by the same orders of magnitude. However, this concerns auxiliary materials which are not incorporated into the product.
For example, the embodied energy of the input cleaning gases in the CVD process (not included in the figures above) is more than 4 orders of magnitude greater than that of the product output. Furthermore, these gases have to be treated to reduce their reactivity and possible attendant pollution. Gutowski writes: “If this is done using point of use combustion with methane, the embodied energy of the methane alone can exceed the electricity input.”
The benefits of digital technology
Microchips also have positive effects on the environment, by making other activities and processes more efficient. This is the subject of a publication by the Climate Group, an initiative of more than 50 of the world’s largest companies. The report ("Smart 2020 - enabling the low carbon economy in the information age") confirms the findings of other studies regarding the electricity use of electronic equipment, but also calculates the benefits.
According to Smart 2020, the emissions from Information and Communications Technology (including the energy use of data centres, which the IEA report does not include) will rise from 0.5 Gt CO2-equivalents in 2002 to 1.4 GtCO2-equivalents in 2020, assuming that the sector will continue to make the “impressive advances in energy efficiency that it has done previously”. By enabling energy efficiencies in other sectors, however, ICT could deliver carbon savings 5 times larger: 7.8 Gt CO2-equivalents in 2020.
Addressing technological obsolescence would be the most powerful approach to lower the ecological footprint of digital technology
These benefits are smart grids (2.03 Gt), smart buildings (1.86 Gt), smart motor systems (970 Mt), dematerialisation and substitution (by replacing high carbon physical products and activities such as books and meetings - with virtual low carbon equivalents such as electronic commerce, electronic government, videoconferencing, 500 Mt) and smart logistics (225 Mt). One of the first tasks of ICT will be to monitor energy consumption and emissions across the economy in real time, providing the data needed to optimise for energy efficiency.
The report concludes: “The scale of emission reductions that could be enabled by the smart integration of ICT into new ways of operating living, working, learning and travelling, makes the sector a key player in the fight against climate change, despite its own growing footprint.”
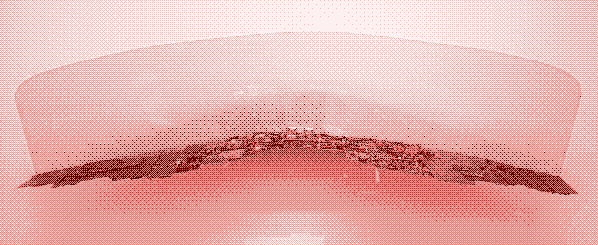
But even if we assume that all these savings will materialise (the report acknowledges that this will not be an easy task), this conclusion does not take into account the energy needed to manufacture all this equipment. If we assume the share of manufacture to be 80 percent of total energy consumption by ICT (following the only life cycle analysis of a computer we have), then the 1.4 Gt in 2020 in reality should be 7 Gt - almost as much as the 7.8 Gt that will be saved by ICT. No environmental benefit would appear and the energy savings realised by digital technology would merely absorb its own growing footprint.
Digital technology is a product of cheap energy
The research of Timothy Gutowski shows that the historical trend is toward more and more energy intensive processes. At the same time, energy resources are declining.
Gutowski writes:
“This phenomenon has been enabled by stable and declining material and energy prices over this period. The seemingly extravagant use of materials and energy resources by many newer manufacturing processes is alarming and needs to be addressed alongside claims of improved sustainability from products manufactured by these means.”
Production techniques for semiconductors and nanomaterials can and will become more efficient, by lowering the energy requirements of the equipment or by raising the operating process rate. For instance, the “International Technology Roadmap for Semiconductors” (ITRS), an initiative of the largest chip manufacturers worldwide, aims to lower energy consumption (pdf) per square centimetre of microchip from 1.9 kWh today to 1.6 kWh in 2012, 1.35 kWh in 2015, 1.20 kWh in 2018 and 1.10 kWh in 2022.
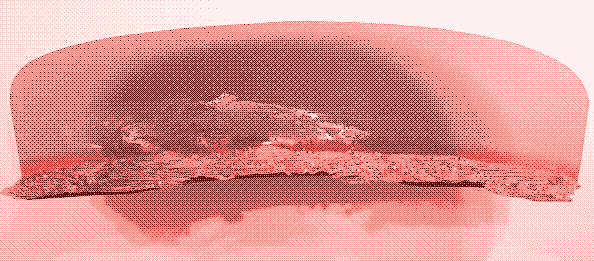
But as these figures show, improving efficiency has its limits. The gains will become smaller over time, and improving efficiency alone will never bridge the gap with conventional manufacturing techniques. Power-hungry production methods are inherent to digital technology as we know it.
The ITRS-report warns that:
“Limitations on sources of energy could potentially limit the industry’s ability to expand existing facilities or build new ones”.
Gutowski writes:
“It should be pointed out that there is also a need for completely rethinking each of these processes and exploring alternative, and probably non-vapour-phase processes”.
Technological obsolescence
The ecological footprint of digital technology described above is far from complete. This article focuses exclusively on energy use and does not take into account the toxicity of manufacturing processes and the use of water resources, both of which are also several orders of magnitude higher in the case of both semiconductors and nanomaterials. To give an idea: most water used in semiconductor manufacturing is ultrapure water (UPW), which requires large additional quantities of chemicals. For many of these issues, the industry recognizes that there are no solutions (see the same ITRS-report, pdf). There are also the problems of waste & war.
Last, but not least: the energy-intensive nature of digital technology is not due only to energy-intensive manufacturing processes. Equally as important is the extremely short lifecycle of most gadgets. If digital products would last a lifetime (or at least a decade), embodied energy would not be such an issue. Most computers and other electronic devices are replaced only after a couple of years, while they are still perfectly workable devices. Addressing technological obsolescence would be the most powerful approach to lower the ecological footprint of digital technology.
Artwork by Grace Grothaus. More information on manufacturing methods.
Reactions
To make a comment, please send an e-mail to solar (at) lowtechmagazine (dot) com. Your e-mail address is not used for other purposes, and will be deleted after the comment is published. If you don’t want your real name to be published, sign the e-mail with the name you want to appear.
Reactions
Jan
Please get your units straight. Watt is a unit of power, not energy. Watt-hour on the other hand is a unit of energy, like Joule. It’s wrong to say “..consume 60 watts of electricity per hour”. Just lose the “per hour” and it’s better already.
Otherwise, another great in-depth article on an important issue.
Thanks!
Jon
So if we figure embodied energy in a product does that me the manufacturing equipment runs for free? Why not just say this manufacturing equipment and processes are inefficient?
kris de decker
Jan: thanks, corrected. Also good to hear that at least someone agrees this is an important issue :-)
Jon: manufacturing equipment and processes are inefficient and can be improved substantially. But energy efficiency has its limits and can never solve this issue by itself (see the article). We also have to address technological obsolescence. If products are so energy-intensive to manufacture, it is insane to replace them after two or three years. With servers and network appliances embodied energy is already of lesser importance, because they are only replaced after 5 to 8 years.
D
Just to add to the energy units.
This bit doesn’t make sense “(around 180 watts, or, using the appropriate unit of measurement, 648 kilojoules)” since the comparison is between rates of energy consumption. Similarly, “Manufacturing one kilogram of electronics or nanomaterials thus requires between 280 kilowatt and 28 megawatt of electricity” doesn’t make sense either here you want to be using amounts of energy rather than rates of use. These were the two errors I noticed, but I think you should go through the article again to check for other instances in what is otherwise a very interesting piece.
kdd
Wow, Gert, many thanks for sharing this.
Gert van Loo
Nice article but how much worse does it get if you add the design energy?
I am one of the engineers designing these chips.
We are a team of 60 people designing about 1 graphics chip per year. For that we use 2/3 building, about 3 managers, 3 sales persons, 4 administrators, 2 IT persons. 2 LCD screens/person. 1 computer/person and computer farm of about 74 very high end Linux boxes (highest possible speed with 16-64Gbytes of memory each) with all the networking file servers etc. etc. all of which run 24/7.
Maybe you could from that info estimate how much more energy is required on top of the production energy.
Jannah
Excellent post. This is the first article that I’ve ever read covering this hidden problem. I recently retired from the semiconductor manufacturing field, and this has been an environmental concern of mine for a long time.
The direct manufacturing alone is astronomically energy consumptive. On the fab line, and in the analysis support labs, the machinery runs 24/7, including vacuum systems, chillers, ovens, SEMs, and all manner of connecting and contributing mechanical systems – all of these equipment components running non-stop every day of the year.
Second on the consumption list is the vast use of support materials, e.g., the water, acids, metals, gases, etc. Not to mention consumables such as cleanroom accouterments. And not only can the direct usage be accounted for, the amount of energy expended for the waste products involved such as disposable safety apparel, chemwipes, oils from vacuum pumps, chemicals, etc., discarded daily is enormous as well.
I just shake my head when I hear someone comment that electronic equipment is so small now that it hardly makes an impact on the environment at all. You are correct in stating that the smaller processors get, the more difficult and consuming it is to manufacture them (and I’ve seen it all the way back to SLT chips). And I’m very surprised that the environmentalists have their heads completely in the sand about this topic, if they’ve ever even thought of it. They worry about smoke-stacks? If they really took a look at the process details of semiconductor manufacturing their heads would explode. It would be very interesting to see a study published on the overall collective costs of a computer from design stage to finished product.
Donovan
I’m researching a state-of-the-art snapshot of green ICT technologies for a university of applied sciences and an ICT R&D cluster and stumbled upon this article. Very, very interesting. Also I’m a huge proponent of Free Software and totally in agreement with your conclusion. Thanks for the great read!
Pangea Joel
Although i did appreciate your article’s breakdown of manufacturing cost of ICT components, the value of the article was severely detracted from by your misleading and fallacious conclusion:
“No environmental benefit would appear and the energy savings realised by digital technology would merely absorb its own growing footprint.”
i just read the Smart2020 report referenced in your article.
http://www.theclimategroup.org/assets/resources/publications/Smart2020Report.pdf
Appendix 1 defines the Climate Group’s methodology of calculating “embodied carbon.”
Appendix 2 clearly does break out explicitly and quantitatively the embodied carbon from the manufacturing processes for the ICT components.
So your concluding claim that the 1.4Gt consumed by ICT in 2020 should be multiplied by 4 and thus reach 7Gt and thus virtually erase almost all the 7.8Gt f energy saving benefits from ICT is fallacious and misleading.
The energy savings of ICT stands.
I am at a loss to understand why you felt compelled to distort the Climate Group’s report you referenced.
Are you so motivated by your ideology that you failed to read the Smart2020 report you referenced?
Or were you hoping that no one would drill down to the source material?
Kris De Decker
Hi Tom,
Thanks a lot for the additional information. Do you know where Vaclac Smil (the author of the book you mention) got his numbers?
It would make sense if the energy intensity would be roughly half my number by now. As I write in the article: “The International Technology Roadmap for Semiconductors (ITRS), an initiative of the largest chip manufacturers worldwide, aims to lower energy consumption per square centimetre of microchip from 1.9 kWh today to 1.6 kWh in 2012, 1.35 kWh in 2015, 1.20 kWh in 2018 and 1.10 kWh in 2022.”
My number of 20 kWh per 2 grams of microchip was based on this information and on the 2002 research of a 32 MB RAM chip (in 2009 still the most up-to-date study available).
However, Smil’s book was published in 2007, two years before my article, and then the numbers don’t correspond. So I wonder to which study he refers.
davea0511
Another way to do this is to find the cheapest competing brand (people pay a lot for a brand name), subtract out all the employee benefits costs, raw material costs, salaries, maintenance, vendor service costs, and profit (without profit companies go bankrupt), and you’ll be left with the total energy cost. Divide that by the cost of energy and you’ll have the kWh burned.
Take the iPhone … cheapest competing product (without a plan) can be had for $200. Subtract all that gunk I mentioned out and I bet the number ends up somewhere around $20, maybe more. At $0.05/kWh that device then took 400kWh. It takes an average household (14MW/yr) 10 days to consume that much energy. By buying that iPhone you just consumed as much energy as an average family uses for 10 days. That’s alot.
K-dude
What about Apple Inc. and their Eco report? The impact of the aluminum unibody manfacturing process and use of renewable energy sources?
Brent Norris
Awesome, let’s go back to building stores out of bricks so we can drive to buy more things! Just kidding, great article, great work. Very comprehensive.
I wonder how energy intensive self-assembled, tablet, 100% powered by the sun will be?
James
I work in a UK science and technology institution, in the ICT department and measured the power usage of the whole department. I worked out that lights, monitors and P.C’s only used about 10% of annual energy consumption, with the energy hogs being fan coil units that run all day, everyday for the entire year.
I think if you use digital technology effectively - Digital cameras, MP3 downloads, webconferencing, it can save energy or use less than conventional methods, but the problem is, the old methods are not becoming obsolete. they are still here.
The biggest problem we have is infrastructure, which is much, much more difficult to alter and make efficient, both practically and bureaucratically.
Jumper
One can simplify matters a great deal by realizing that your $700 computer did not take more than $700 of energy to make. That would be your conservative, worst case scenario.
Ivor Cogdell
A very good article. To extend the life of Motherboards, use a modular, plug in, approach to all micro-processors, not just the main one. All internal and external connections should be standard (not always the case, in my experience). To extend the usage of individual memory cards, design them with a memory slot on top, so another one can be plugged into it when needed.
I think computer recycling should be given much more publicity.
Leonardo
Hello, excellent article.
After the financial crisis following 2008, many people started finally understanding that infinite growth is not sustainable, that the principle on which our economy stands are not acceptable anymore and so on (I hope you already have heard of this, in Italy it is a big topic now).
I was wondering about the 2020 estimate: in 2020 the ICT will save 7.4Gt. Now, EVEN IF your assumptions or data were not correct, and thus say that ICT costs a bit less than 7Gt you calculated, say 5Gt obtaining an overall saving of 2.4Gt by 2020. EVEN IF it saves something on the short term, market laws require infinite growth! It means that then, say 10 years later, the global market has doubled, thus the ICT costs 10Gt CO2 and saves 4Gt. They may say “we doubled our CO2 saving”, but they actually doubled the CO2 emissions: 14Gt. What I mean is that the 2020 report is not only deceiving (I didn’t read it, but I understand from the comments that references were bad), but also ridicoulus: if the market requires growth, the environment can never be saved.
What do you think about that?
Adrián A. García
First of all, congrats on this blog, it is really fantastic.
Now to the point. Perhaps an energy-saving solution for our everyday computing needs could be computers able to do most of what we usually do with them but at the same time using a fraction of the materials and energy that we currently use on a typical computer. And by this I mean a truly significant reduction of them.
Take the Raspberry Pi as an example: http://www.raspberrypi.org/faqs. A 25$ computer with the size of credit card made of very few electronic components and able to do multimedia tasks. In time more capable computing platforms based on the Raspberry Pi philosophy will surely arise, rendering our current PC solutions obsolete.
What I try to say is that if people becomes aware of how important it is to reduce the energy footprint of electronic products, great progress could be made in a relatively short period of time. Because, let’s be realistic here, it’s doubtful that people suddenly stops buying and using electronic appliances, most of them designed from the beginning to last no more than their warranty.
CJLindy
Here’s my thing. To me it’s misleading when you compare the energy to manufacture of heavy simple “normal” goods/lb, vs. Electronics/lb. You get all of these figures, but no indication of the “Utility”/KWhr.
Keeping electronics (or any thing) longer is an excellent idea, it is antithetical to our Marketing driven economy. How would anyone accomplish the same utility of a computer (Word processing, publishing, design, etc.), with some “normal” low-tech device?
While the Moon was reached with mostly slide-rules and T-squares, almost every goods/articles in use today would be UNPRODUCABLE with those limitations.
In Summary, to get tens of orders of Magnitudes of savings, it would be much better to reduce population - the real driver behind our profligate energy consumption.
Tom M
We could also estimate maximum embodied energy by manufacturing cost.
“A 16GB iPhone 5S costs Apple Inc. $213 in materials, while a 16GB 5C comes in at $156, according to a report published Monday by UBS AG.”
213$ assume that was pure energy usage -> .12$/KWh ->
1775 kilowatt hours for the most expensive iPhone.
http://blogs.wsj.com/tech-europe/2013/09/30/how-much-does-it-cost-to-make-an-iphone/
Or the 25$ raspberry pi above 25$ * 1 KWh/.12$ -> 208 KWh
Manu Sharma
Apple publishes life cycle energy analysis for each device and if I remember correctly the manufacturing and usage energy ratio for Macbook was 60:40. If that’s correct than the claim about embodied energy for memory chip might not be correct. The data should still be on Apple’s site.
Francesco Pasa
I think the main problem is obsolescence, and that is a huge problem to solve, because the entire IT market revolves around selling new products when the old ones are just good enough for the same tasks. Software is also big part of the problem, because most programs become heavier on the hardware every year (especially OSes and browsers).
I believe the solution is rather simple: (1) Design software to run on old hardware (or low-power like the raspberry) and support it for longer, so that we can use the same hardware for longer. (2) Make hardware modular and replaceable. If my laptop’s processor could be changed, I would use it for 10 years instead of just 5 (and I believe I’m one of the few who use a computer for ‘so’ long), saving the rest of the hardware. Make broken parts easily replaceable. That is an issue also for the raspberry, which is a one-piece board with all component surface-mounted. You cannot adapt your hardware by just replacing the needed part.
I think the problem is more one of mentality (to be cool you need the latest gadget) and of market.
Have you tried looking on the apple website? Mike Bernares-Lee in his “How bad are bananas?” writes about a life-cycle assessment report from apple on his products.
Great article, keep up!
Jonathan Sundqvist
There are actually two LCA industry studies, one done by dell: https://www.one-report.com/download.html/2011/shared/library/0692-00006713.pdf and then LCAs done by apple (http://www.apple.com/environment/reports/). The carbon footprint of a laptop is between 300-400 CO2eq and manufacturing represents between 50-65% of that footprint. So your number of 80% is way off to be frank.
You might be interested in reading this too: http://greenelectronicscouncil.org/wp-content/uploads/2013/12/slateswkshp/GECTabletsWorkshopDec2013_LCA_Teehan.pdf
Efried
I think we should research LCA of quickly depreciating goods and parts:
batteries, in remote controls etc..
accumulators, now that smart phones and watches need daily charging, and we have electric toothbrushes etc..
SD cards, USB memory sticks- not only electrical wear but also mechanical damages increase turnover
embedded electronics (greeting cars, wearables…)
headphones
….
Vikas Kashyap
A decent article. To expand the life of Motherboards, utilize a particular, connect to, way to deal with every single miniaturized scale processor, not only the principle one. All inward and outside associations ought to be standard (not generally the situation, in my experience). To broaden the use of individual memory cards, plan them with a memory opening to finish everything, so another can be connected to it when required. I think PC reusing ought to be given substantially more attention.
yeen
I think the most drawback is degeneration, which may be a vast drawback to unravel, as a result of the whole IT market revolves around commerce new product once the recent ones square measure simply ok for constant tasks. software package is additionally massive a part of the matter, as a result of most programs become heavier on the hardware each year (especially OSes and browsers).
I believe the answer is quite simple: (1) style software package to run on recent hardware (or low-power just like the raspberry) and support it for extended, in order that we will use constant hardware for extended. (2) create hardware standard and replaceable . If my laptop’s processor might be modified, i might use it for ten years rather than simply five (and i feel i am one in every of the few UN agency use a laptop for ‘so’ long), saving the remainder of the hardware. create broken elements simply replaceable . that’s a difficulty additionally for the raspberry, that may be a one-piece board with all element surface-mounted. you can’t adapt your hardware by simply commutation the required half.
I think the matter is additional one in every of mentality (to be cool you would like the most recent gadget) and of market.
Have you tried counting on the apple website? electro-acoustic transducer Bernares-Lee in his “How unhealthy square measure bananas?” writes a couple of life-cycle assessment report from apple on his product.
Great article, keep it up!
Kudos…