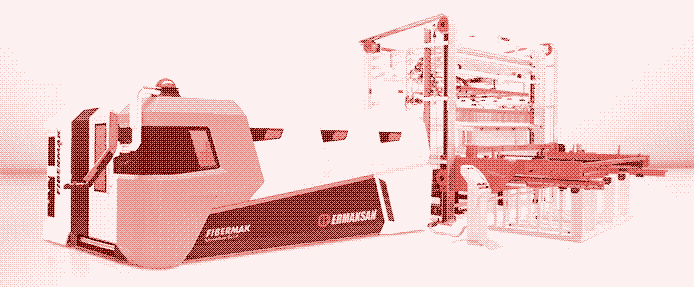
.
Digital fabrication is praised as the future of manufacturing. Computer Numerical Controlled (CNC) machine tools can convert a digital design into an object with the click of a mouse, which means the production process is completely automated. The use of digital machine tools has spread rapidly in factories over the last two decades, and they have now become cheap and user-friendly enough to bring them within reach of workshops and makers.
While CNC machines have been embraced by many, including some environmentalists who say the technology can be more sustainable, it’s important to consider the very high energy use of digital machine tools. Compared to the earlier generation of human-controlled machine tools, CNC machines use much more power, and the potential to improve their energy efficiency is very limited. Choosing fewer automated technologies is the key to sustainable manufacturing.
We’ll create even more stuff, and each product will cost much more energy than if produced with conventional methods.
Manufacturing processes can be broadly divided into two groups: primary and secondary. The former — such as forging and casting — provide basic shape and size to the material. Secondary processes provide the final shape and size with tighter control on dimension and surface characteristics. This second group is performed by so called “machine tools”.
Machine tools form the basis of precision manufacturing. As such they are indispensable for the production of nearly all modern technology, be it cars, planes, household appliances, computers, solar panels, wind turbines, or other machine tools. They are so fundamental to manufacturing that the United Nations Industrial Development Organization (UNIDO) defines “industrialisation” as the capacity to produce machine tools. 1
Machine tools are stationary operating machines that perform the geometric shaping of workpieces. 2 They are mostly subtractive technologies, dealing with any process in which material is removed gradually from a workpiece. There is a great diversity in specialized machine tools, but common examples are sawing and cutting machines, drilling machines, grinding machines, turning machines (lathes) and milling machines.
Machine tools can process different materials, but today most of them are used for the shaping of metal parts. Milling machines have become the most common metal shaping tools in the mechanical manufacturing industry. Milling is a process of cutting away material by feeding a workpiece past a rotating cutting tool. A milling machine operates like a human sculptor. It can have grinding, cutting and polishing heads.
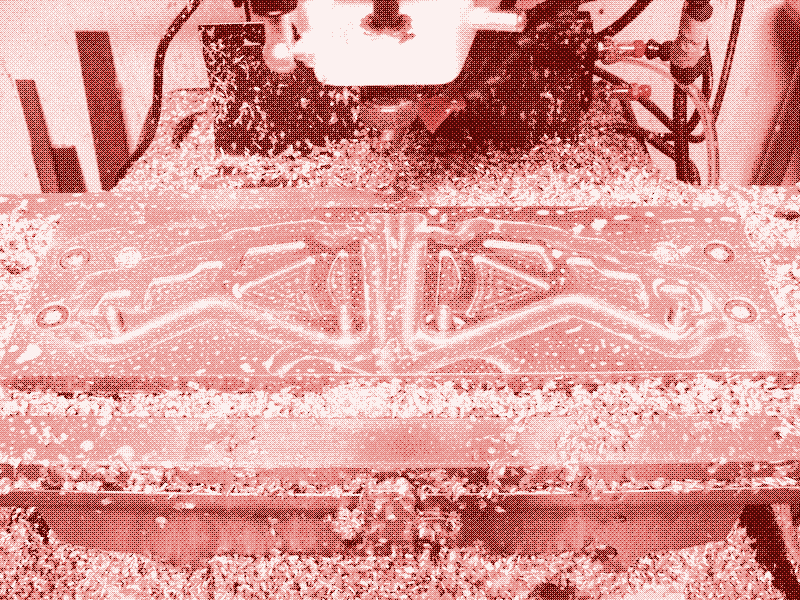
Lathes or turning machines are similar to milling machines but they are used to shape cylindrical workpieces. In a milling machine the workpiece remains (relatively) stationary while material is removed by moving tools heads. In a lathe, the workpiece is rotating while the tool head remains (relatively) stationary.
How to Power a Machine Tool
Modern definitions of machine tools exclude any machine that is powered by humans. However, the predecessors of most machine tools were hand- or foot-powered. Woodworking hand tools with the same kinematic motions as modern machines, such as the cord lathe and the bow drill, were developed in ancient times. 3
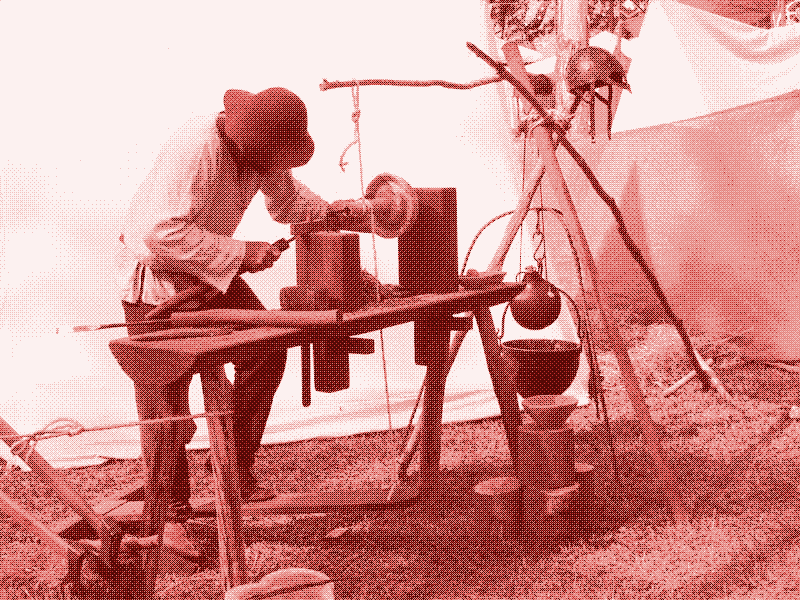
More sophisticated, foot-driven wood lathes, which had many of the essential features of modern turning machines, appeared in the middle ages. 4 Lathes for metalworking, which were originally developed for the production of helical threads, emerged in the 1700s. 5 The first real milling machine for metalworking was built in the early 1800s, although similar operations were performed on adapted lathes by clockmakers at least one century earlier. 5634
Wind and water-powered mills from pre-industrial times fit the modern definition of machine tools
Machine tools were also driven by animals, wind or water power. The Romans already had water-powered sawmills for cutting marble, but most automatic machines only appeared in the middle ages. The Europeans used sawmills, boring mills, turning mills, grinding and sharpening mills, polishing mills, and metal slitting and rolling mills that were powered by water, wind or animals. 7 8
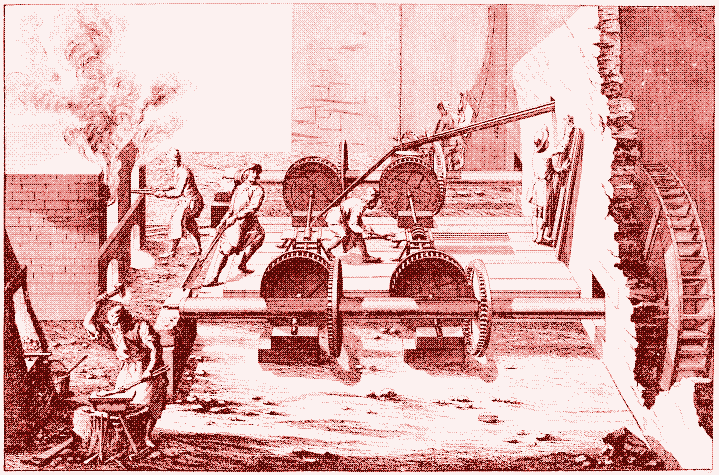
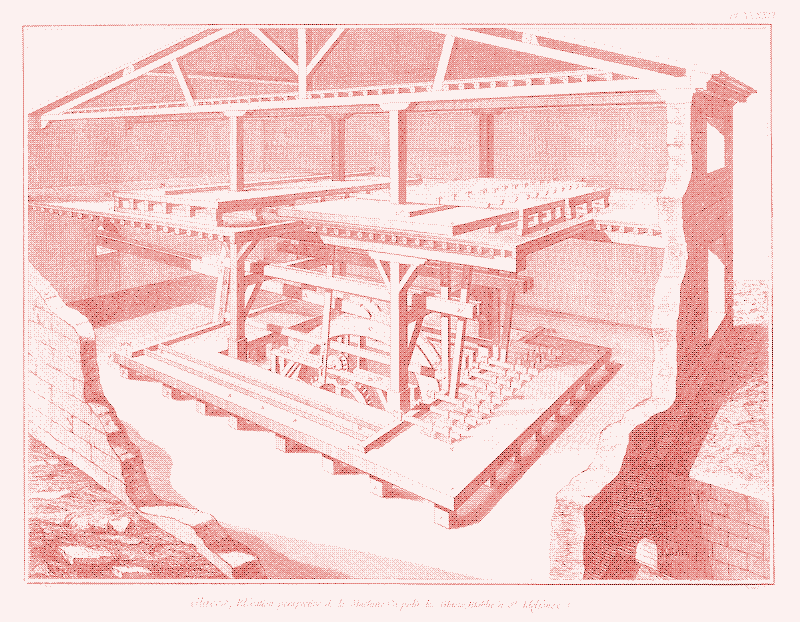
These industrial mills were stationary, complex machines using non-human power sources, and thus fit the modern definition of machine tools. However, from the 1850s onwards, machine tools were increasingly powered by steam engines, and today almost all machine tools are powered by electricity.
How to Operate a Machine Tool
The evolution of machine tools continued throughout the twentieth century, though most improvements were now made to the operation of the process. During the first half of the century, all machine tools were hand-operated. The cutting tool itself was powered by steam engines or electricity, but workers shaped the workpiece and took care of all auxiliary actions.
For example, in a milling machine, motors only ran the drill and mechanized the x-axis bench movement. 9 All other movements were initiated and controlled by the operator, one at a time, often by use of hand wheels. 10
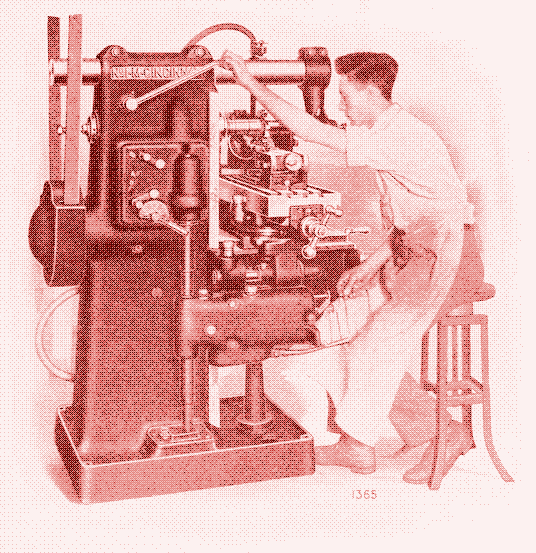
These so-called “manual” machine tools are still in use, but they’re becoming increasingly rare. In the early 1950s, the first automatic machine tool was developed. Data was fed into the machine on punched tape, which allowed the machine tool to work independently. These “numerically controlled” (NC) machine tools became commercially available in 1960. 4 Around 1980, tape control was replaced by computer control, which led to the development of “computer numerically controlled” (CNC) machine tools.
Until the 1980s, most machine tools were controlled by humans. The tool itself was powered by electricity, but workers shaped the workpiece
CNC machine tools heralded the era of digital manufacturing. With CNC machines, human input is limited to the creation of a digital design on a computer, which is then automatically converted into a physical object by the machine tool. To achieve this, the digital design is fed into software that converts it into a set of symbolically coded instructions arranged in a proper sequence. The computer of the CNC machine then executes the program step by step by moving the machines slides and the tool head accordingly. 411
Increasing Automation
Most machine tools built after 1985 are CNC machines, but considering the long life expectancy of machine tools means the switch to CNC machines is still on-going. 3 For instance, in 1995, only 350,000 of the 3.5 million metal-working machine tools in Europe were CNC machines. In 2009, this number had increased to 750,000. 12 At the same time, the unit value of CNC machine tools raised significantly from 1995 to 2009, indicating that the machines have become more complex. 12
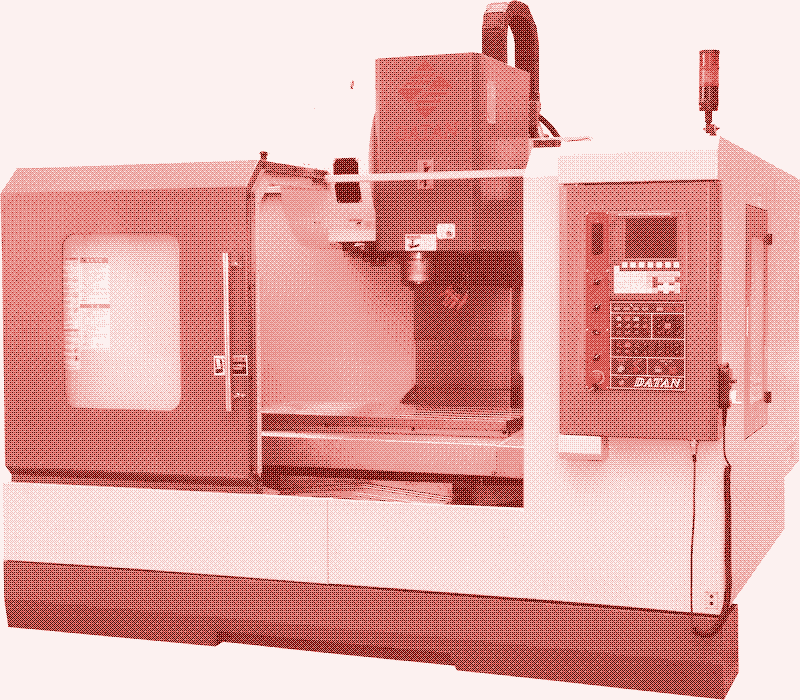
The level of automation in a CNC machine can vary considerably. For example, in a milling machine, apart from the positioning and powering of the tool, auxiliary functions such as machine lubrication, tool changes, chip handling, tool break detection, or the loading and unloading of workpieces can all be set in automatic code, but they can also be operated by hand. 411 The trend, however, is a move towards more automated auxiliary functions. 13
Automation also makes it possible to design more complex machine tools. There are now milling machines with four, five or more axes which also support rotation around one or multiple axes, while 3-axis milling machines can only cut away material horizontally and vertically.
Power Use Increases with Automation Level
It’s obvious that the switch from human- and water-powered tools to fossil-fuel powered tools has made manufacturing less sustainable. What’s surprising, however, is that the switch from human-controlled machine tools to computer-controlled machine tools has much larger consequences for energy use. Automation is more energy-intensive than mechanisation.
A computer-controlled milling machine requires 2.5 to 60 times more power than a hand-controlled milling machine
A comparison of the maximum power requirements by three CNC milling machines (from 1988, 1998 and 2000) and one hand-operated milling machine (from 1985), all cutting a similar workpiece, revealed that the digital machines require 2.5 to 67 [^sic] times more power than the manual machine. At full operation, the hand-operated machine tool used 2.8 kW, while the digital machines used 7 kW for the 1998 machine, 9.4 kW for the 1988 machine, and 188 kW for the 2000 machine. 13[ ^9]
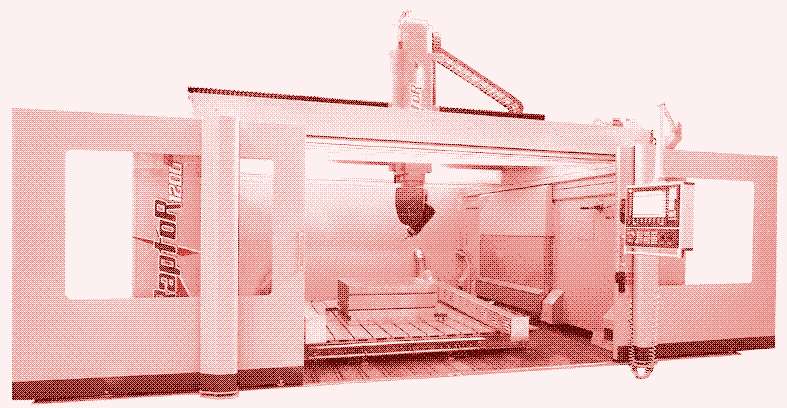
.
The authors of this widely cited paper, Jeffrey Dahmus and Timothy Gutowski, show that the large difference in power use between several types of CNC milling machines is mainly due to different automation levels. The 2000 machine, which operates in a car factory, is fully automated, while the other two digital machines only have their basic milling operations controlled by computers.
Load-Independent Power Use
The power use of a machine tool is distributed among various activities. Constant start-up operations refer to the load-independent power use of the machine tool. This power is required whenever the machine is turned on, independent of whether or not a part is produced. Run-time operations include the power required for positioning the workpiece and loading the tools. Material removal operations refer to the actual power involved in cutting. 13
![Image: Energy analysis of four milling machines. Source: [^13]. Click to enlarge.](https://solar.lowtechmagazine.com/2014/03/how-sustainable-is-digital-fabrication/images/dithers/energy-analysis-of-four-milling-machines_dithered.png)
In the case of the most automated CNC milling machine, the power used handling the workpiece and tools is less than 15% of the total power required. The remaining 85% of the power used by the machine is constant, even when no action takes place. 13 For the other machines, this share is lower, but still significant. In absolute figures, power use for constant start-up operations (= idling) is 166 kW for the most automated milling machine, 1.3 kW to 3.4 kW for the less automated milling machines, and 0.7 kW for the manual machine. 13 14
Up to 85% of the power used by a CNC machine is constant, even when no action takes place
In all four of the milling machines analysed by Dahmus and Gutowski, the power required to actually cut the material is the same, assuming operating parameters, materials properties, and tool characteristics remain constant. 13 If the material removal rate would be the same for each machine, milling a similar part on a manual machine would take 2 to 5 times less energy than on the least automated CNC machine tools, and 240 times less energy than on the most automated CNC milling machine.
Material Removal Rate
Operating parameters are not the same, however. The material removal rate is 3 to 13 times higher on the more automated machines. 15 Because energy consumption equals power consumption multiplied by time, in the end a CNC machine might use less energy than a manual milling machine for the processing of a similar part.
Higher production rates also increase the power consumption at the tool tip: from 2 kW for the manual machine, to 6 kW for the least automated CNC machines and to 22 kW on the fully automated CNC machine. 13 Higher production rates require stiff mechanical systems with the capability to absorb arising inertia forces. As a consequence, the masses of the machine structure, such as moving machine components, have to be increased. This, in turn, require motors with high torque output which are able to increase the forces needed during acceleration and deceleration. 16
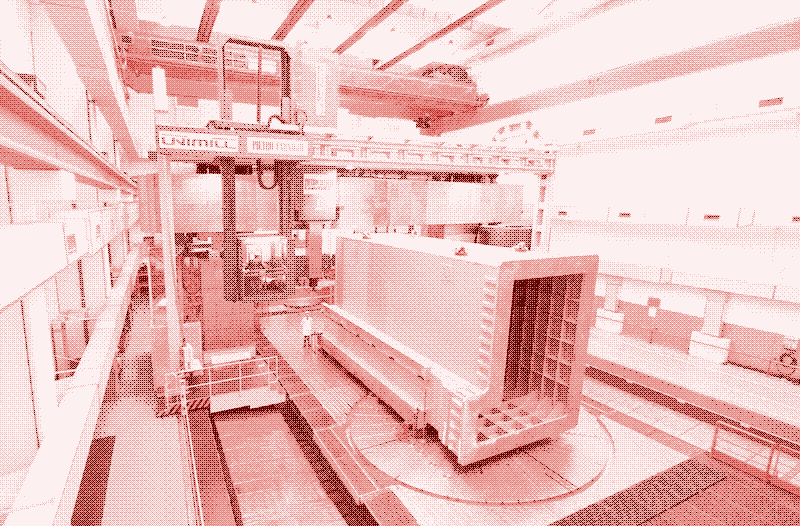
On the other hand, because of a higher production rate, the high power required for maintaining the CNC machine tools in a “ready” position can be divided over a larger amount of parts. If you calculate the energy used per unit of material removed, then the two less automated CNC milling machines have a similar efficiency as the manual machine. Assuming 1,000 work hours (30% of machine time positioning, 70% of machine time cutting, and 0% idling), the human controlled machine requires 6.2 kJ of energy to remove one cubic centimetre of material, while the CNC milling machines require 4.8 to 7.1 kJ of energy. 13
The fully automated milling machine, on the other hand, requires 39 kJ per cubic centimetre of material removed, and is thus at least five times less efficient per unit of material removed than the other machines. 13
Idling Time
An idling time of 0%, as we have assumed, is not realistic. In fact, machine tools are rarely switched off between jobs and often remain idling even at night, notes the German Fraunhofer Research Institute in a 2012 study on sustainable manufacturing methods:
“There are various reasons why machine tools are frequently not shut off at the end of a shift: One reason is to ensure thermal steady-state conditions with a specific thermal strain, which is very important for high accuracy of metal working machine tools, complex products and work pieces. Overnight shut off or other periods of non-operation of machine tools could result in processing temperatures to fall below desirable levels, and thus having an adverse impact on the process and manufacturing precision.” 17
When idling times increase, the energy use per cubic centimetre of material removed increases significantly for digital machines, and much less so for the hand-operated machine tool. If the two least automated milling machines are kept idling all night, their specific energy consumption would be much larger than that of the manual machine. This would be even more so for the fully automated CNC machine, but Dahmus and Gutowski note that these types of machines are often operated 24 hours per day and are idling less than 10% of their total life time. 13
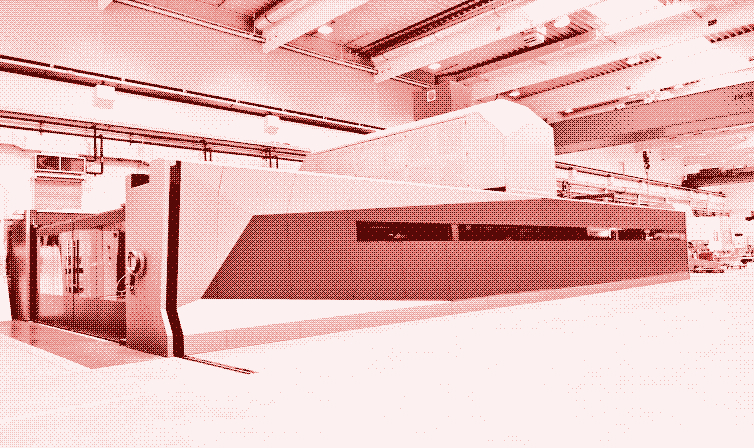
Easily Solved?
In theory, there are many opportunities to improve the energy efficiency of machine tools. For instance, engineers have designed alternatives to coolant and lubrication systems, as well as kinetic energy recovery systems. Further energy savings could be achieved by using lightweight but strong materials, more efficient motors, more flexible machines, a transition to power saving modes during stand-by periods, and so on. 181619
However, the energy savings potential of these technologies seems rather small. According to the Fraunhofer Institute, “there is no single option with a large environmental improvement potential, and moderate savings of 3-5% can be realised only with the implementation of several individual options”. For metal working machine tools, they estimate the savings potential at 4%." 20
The incentives to improve the energy efficiency of machine tools are rather small, especially when energy saving measures hamper productivity
The problem is not just technological. The main obstacle is the extent to which machine tool builders are willing to integrate energy-saving technologies. Few manufacturers have used the best available technologies. 2 Kinetic recovery systems, for instance, although considered a big trend in the 1990s, were never broadly introduced into the market. 16
Sustainability versus Productivity
Incentives to improve the energy efficiency of machine tools are rather small. Firstly, energy saving measures may impede productivity. For instance, when taking into account the large power use during idling, it’s obvious that a power saving mode during stand-by periods could be as useful on a machine tool as it is on a laptop. However, the European Association of the Machine Tool Industries notes that:
“Productivity is severely hampered, if too short transition periods from any processing mode to a sleep/standby-mode of the machine tool or parts thereof are made obligatory: warm up and bringing back the machine to full operation state delays the processing”. 21
Secondly, depending on the automation level, energy costs are only 1 to 6% of total machining costs. 2217 A study investigating energy and cost-efficiency in CNC machining concludes that “the energy prices of today are not high enough to pose any particular need for making radical energy savings for CNC machining”. Instead, the researchers advice that “significant cost savings can be achieved if the production output is increased as a consequence from higher removal rates…. If production output can be increased, cost and energy savings can follow”. 22
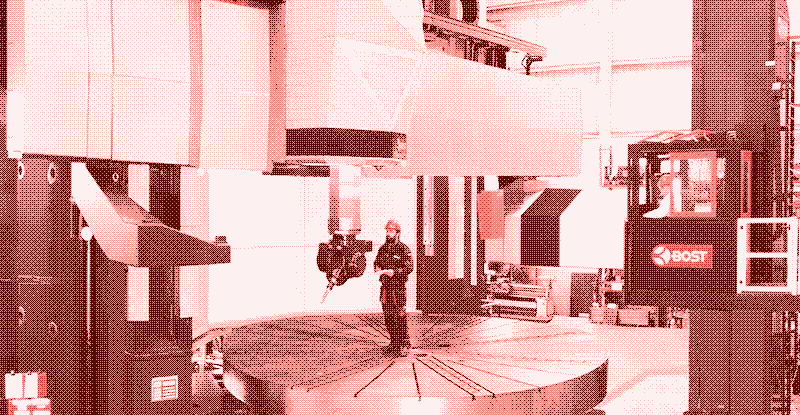
Larger cost savings can be achieved by increasing the production rate rather than by using more energy efficient machine tools, so there is little to win by concentrating on energy use — especially when energy saving measures are in conflict with higher production rates.
Increasing the Production Rate
Further increasing the production rate of digital machining tools will save money, but energy savings are less certain. As we have seen, power use increases as the material removal rate does. The more fundamental problem, however, is that an increase of the production rate also implies an increase in material use.
A machine that works ten times faster also produces ten times more parts and needs as much more raw materials. And that’s detrimental from an energy-saving perspective, because material production completely dominates industrial energy consumption. For example, the manufacture of a one kilogram steel part takes approximately 3 MJ for milling and 27 MJ for steel production. 11
Increasing the production rate of machine tools lowers the specific energy used per produced part. The problem is that it implies an increase in material production, which increases total energy demand.
Lowering the specific energy consumption of CNC milling machines by increasing the production rate thus leads to an important increase in total energy use. This effect would not exist if one CNC machine replaced four manually operated milling machines and total production would remain at the same level. But that’s not the case. CNC machine tools are used precisely because they can produce higher quantity of components in a shorter time and at lower costs. 13
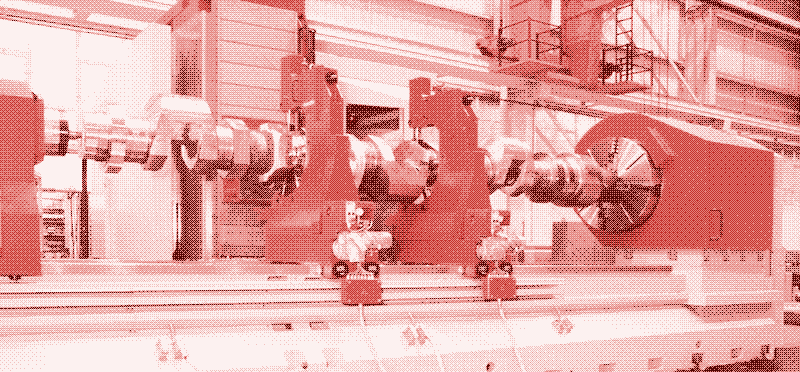
For a given operation, the machining time was reduced from 100 minutes at the beginning of the twentieth century to one minute using modern CNC machine tools. 23 There is still considerable scope for higher production rates: according to a forecast, the future upper limit for the cutting speed of a milling machine, based on the progress in cutting tool materials, will approach one Mach, or 340 metres per second. 23
Non-Conventional Machining Technologies
The rise of CNC machine tools is not the only high-energy trend in manufacturing technology. At least as important is the emergence of so-called “non-conventional” machine tools. Conventional machining processes (such as milling and turning) remove material by applying forces on the material with a cutting tool that is harder than the material. Such forces induce plastic deformation within the workpiece leading to shear deformation and chip formation. 24
Many non-conventional machine tools rely on thermal processes instead; electrodischarge or spark erosion machining (an alternative to milling), laser beam machining, plasma arc machining, and electron beam machining (all alternatives to cutting). Other non-conventional machine tools rely on mechanical processes that don’t use shearing as their primary source of energy, such as ultrasonic machining (an alternative to milling) and waterjet machining (an alternative to cutting). For example, waterjet machining uses mechanical energy, but material is removed by erosion. 25 Yet other non-conventional machine tools are based on chemical or electro-chemical processes. 26
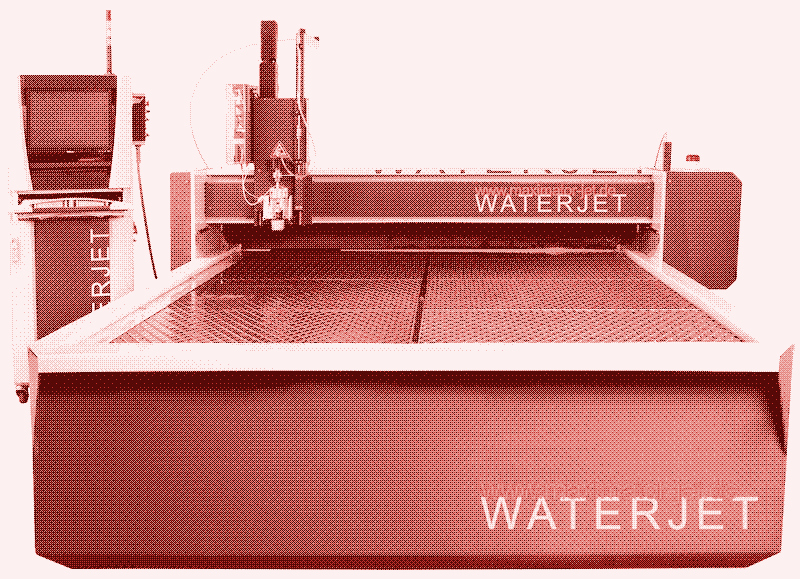
Originally, non-conventional machine tools were developed specifically for workpiece materials that are difficult or impossible to shape using traditional processes. These can be stronger materials of high strength, or materials with high abrasive wear. In the first case, conventional machining is inadequate because it’s time-consuming and leads to unacceptable tool wear. In the second case, conventional machining falls short because the tool bit can cause surface cracks and residual stresses in brittle materials. 26
Laser Cutters
However, some non-conventional machine tools have found extensive use in machining of common materials, because they offer added benefits to traditional machine tools. 26 This is especially true for the laser cutter, which uses a highly concentrated energy beam. Contrary to milling, a three-dimensional process, laser cutters operate in only two dimensions. They have become popular for cutting common sheet metal, at the expense of more traditional technologies such as punching, blanking, and guillotining. 11 Metal cutting machines are the second most important machine tools in the manufacturing industry, after milling machines.
Laser cutters have become popular for cutting common sheet metal at the expense of more traditional technologies such as punching, blanking, and guillotining
Compared to conventional machine tools, laser cutters offer a higher degree of precision and quality of finish. They can create more complex profiles and finer details. Cutting and drilling can be achieved with no force applied to the workpiece, avoiding damage. There is only a small heat-affected zone, which reduces the chances of warping the material (a problem with machine tools based on thermal energy). 26274
Unfortunately, laser cutters have one big disadvantage: they need a lot of power. Being CNC machines, they require substantial power for computer control and auxiliary functions. Apart from that, they also make use of a more energy-instensive cutting “tool” than the machines they replace. A laser cutter works by melting or evaporating material, which is energy-intensive compared to shearing or punching.
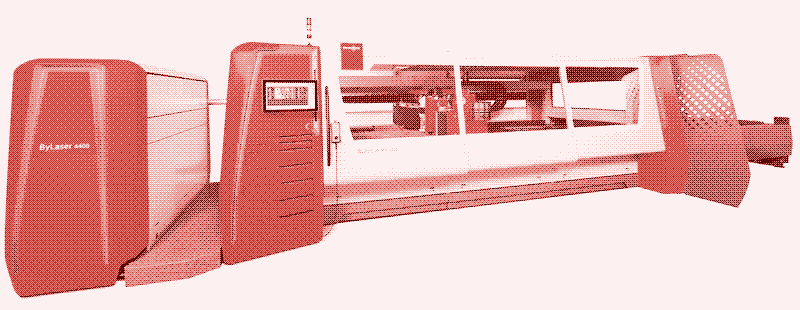
.
This means that laser cutters use even more power than CNC machines. Research has shown that a CO2 laser cutter with a 4 kW laser beam output requires 55 kW of power cutting steel plates at maximum output power, while a modern CNC shearing machine (a “guillotine” cutter) with a similar cutting force requires less than 16 kW of power at maximum output. 1828
Nobody has bothered to measure the power use of a hand-controlled (electrically powered) shearing machine, but looking at power requirements of similar machine tools, this must be less than 5 kW. 10 Even more efficient is a hand-powered shearing machine — still for sale — which requires no external power at all. As with 3D milling machines, we see an evolution in 2D metal cutting towards increasingly power-intensive manufacturing processes, and the increase in power use accelerates over time.
Punching Machines
When used for cutting sheet metal, laser cutters are most often used for making complex shapes instead of straight cuts. This makes them an alternative to punching machines, rather than to shearing or guillotining machines. Just like a laser cutter, a punching machine can cut free forms in 2D sheet metal through the process of nibbling, but compared to laser cutters they have lower resolution and produce parts with a rougher finish.
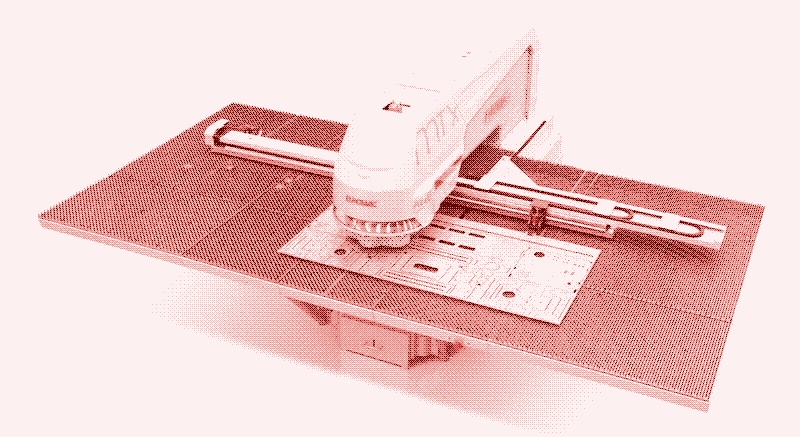
.
Nibbling requires much less power than laser cutting. To my knowledge, the power use of punching machines hasn’t been researched, but the maximum power requirements indicated by manufacturers are between 6 and 11 kW. 29 A CNC punching machine thus requires at least five to ten times less power than a laser cutter.
I also found power requirements for several manually operated nibbling machines in a 1980s catalogue, which are between 1.5 kW (without digital readout) and 2.2 kW (with digital readout). 30 If we would produce a similar part on each of these machines, using a manual machine could thus be 25 to 35 times more efficient than using a laser cutter.
A human-controlled punching machine is 25 to 35 times more efficient than a laser cutter when producing a standard part
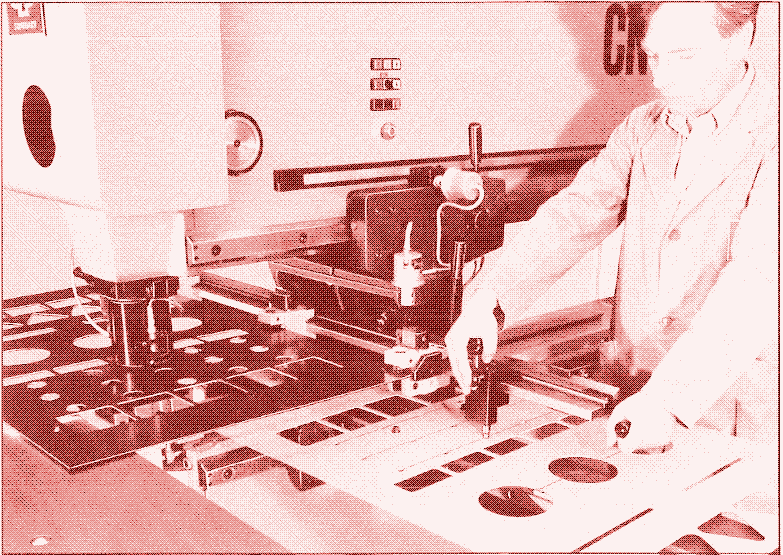
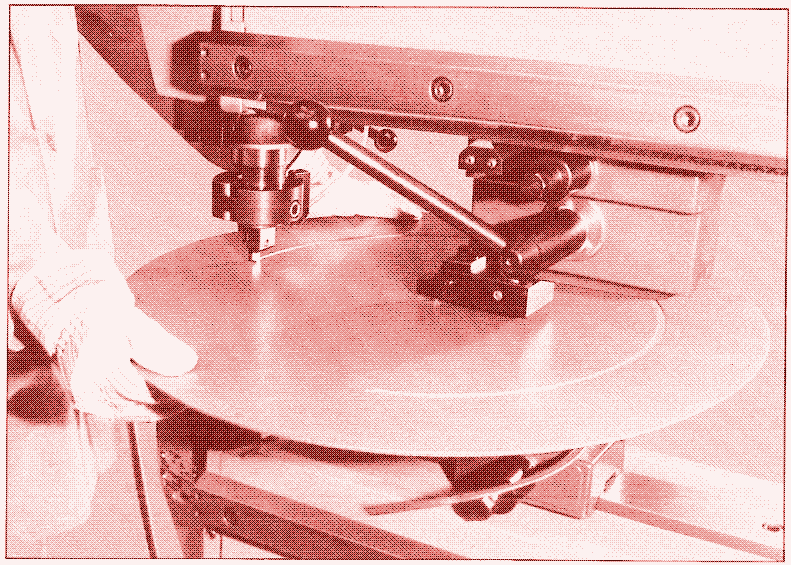
Working speed
Higher power consumption can result in lower energy use per part if the production rate is high enough, but there seems to be no comparison of the energy use of laser cutters and CNC punching or shearing machines available. Figuring out the production rate of these machines is complicated, because it depends on many factors: material properties, material thickness, design complexity, positioning system, and so on.
Nevertheless, from the scattered data I could gather, it seems unlikely that laser cutters are fast enough to compensate for their higher power requirements for most metal cutting jobs. CNC shearing machines are faster than laser cutters for cutting straight lines, and CNC punching machines are faster than laser cutters for many common metal-cutting jobs. The unique selling point of the laser cutter lies less in speed and more in accuracy, product quality, and flexibility.
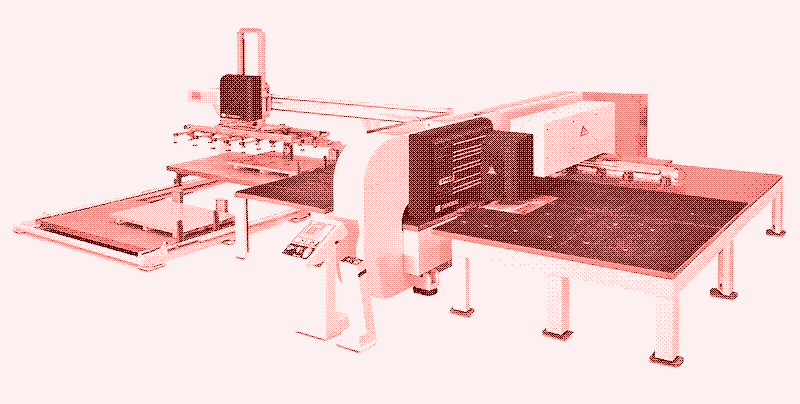
.
A crucial advantage is that a laser cutter needs just one tool, no matter how complex the design of the workpiece. On a CNC punching machine you need to load the right selection of tools for each specific design, and possibly introduce tool changes along the way. This means that the more complicated the design is, the faster and more advantageous a laser cutter becomes compared to a punching machine. This is even higher in small production runs, because there is no need to set up tools.
Laser cutters are thus especially suited for complex quality parts in small batches. This market will grow because as laser cutters spread they will raise the standards of production. Precisely because of the availability of laser cutters, products will get more complex, more accurate, more qualitative, and more flexible.
Fibre Laser Cutters
For laser cutters, energy costs account for about 25% of total production costs. 10 This means that there is more impetus to develop energy-efficient technology, and addressing the power use of the laser source is most profitable. While the CO2-laser is the most commonly used technology in sheet metal industry, the more efficient fibre laser source technology is an emerging alternative. Measurements indicate that a 2 kW fibre laser draws 17.6 kW at maximum laser output, which compares to 33 kW for a 2.5 kW CO2-laser and 59 kW for a 4 kW CO2-laser. 18
The maximum power requirements of fibre lasers are thus about two to three times lower than those of a CO2-laser. This comes close to the maximum power use of a CNC shearing machine (16 kW), but it is still double that of a CNC punching machine, and about 10 times higher than the power output of a hand-controlled (electrically powered) nibbling machine.
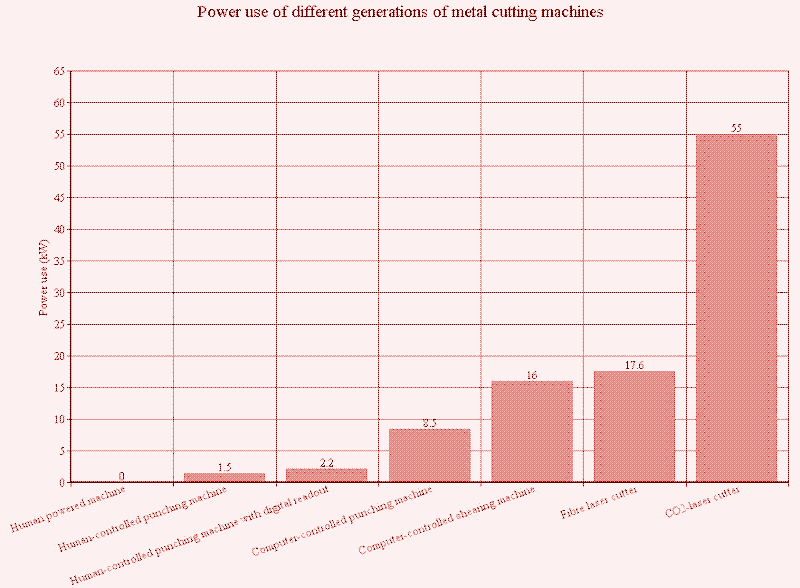
Fibre laser cutters are more efficient than CO2-laser cutters, but their power consumption is still 10 times larger than that of a slower but equally powerful human-controlled nibbling machine
Furthermore, fibre laser cutters cannot replace CO2-laser cutters for all applications, at least not yet. The technologies complement each other. For sheet metal thicker than 4 to 6 mm, CO2 lasers are still the best choice regarding energy efficiency, working speed and cutting quality. 10 20 18 Fibre lasers cannot cut wood or acrylics, but they can cut brass and copper. For CO2-lasers, it’s the other way around.
Manufacturing Becomes Increasingly Energy Intensive
By looking at two of the most important machining processes — metal milling and cutting — it becomes clear that our manufacturing methods are becoming more energy-intensive. This is remarkable, because there are many reasons to believe that we should move in the opposite direction. Our dependence on non-renewable energy sources has created tough challenges, like climate change, peak oil, safekeeping nuclear waste, environmental degradation, and geopolitical conflicts. If we plan a switch to a manufacturing industry based on renewable energy, we would be smart to evolve to less energy-intensive machine tools instead.
Automated machine tools can never become as energy efficient as their hand-controlled counterparts. Replacing human operators requires energy. A fully automated machine will always consume more energy than a semi-automated machine. So, choosing fewer automated manufacturing technologies should be at least part of the solution.
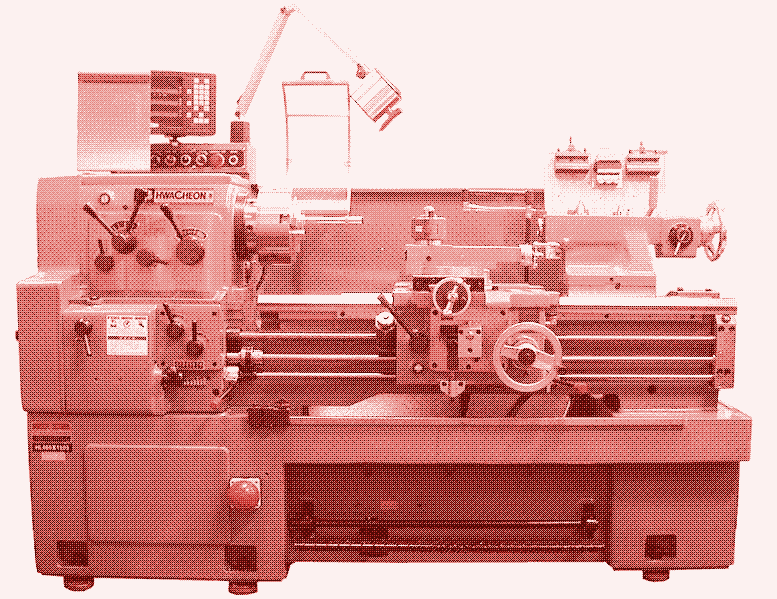
Choosing less automated manufacturing technologies should be a part of the solution
Energy use is not the only concern. CO2PE, an initiative that aims to coordinate international efforts to document, analyse and improve the environmental footprint of manufacturing processes, notes that:
“The intensifying use of non-conventional processing techniques, such as electro-chemical and laser based processes, results in the generation of emissions that have hardly been investigated from an environmental perspective. These undocumented and hard to control material flows are likely to imply significant potential human health hazards.” 31
CO2PE and similar research efforts do not aim to promote fewer automated machine tools. Instead, they aim to promote making automated machine tools as efficient (and safe) as possible. This strategy will spur economic growth, but won’t prevent the increase of energy consumption in manufacturing. While energy efficiency improvements will be realized, Dahmus and Gutowski note that “efficiency improvements in auxiliary equipment may in fact lead to increases in the sales of auxiliary equipment.” 13
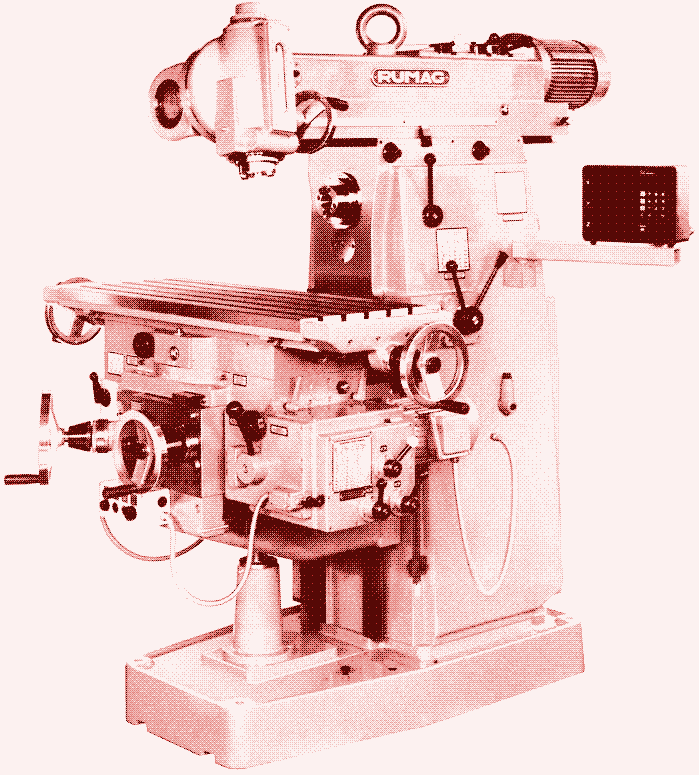
Manufacturing technology is a posterchild for the paradox of energy-efficiency. 32 The more efficient digital machines become, the more they replace older generations of machine tools and find new applications, and the larger their influence in total energy use will be. Likewise, improvements in energy-efficiency pave the way for ever larger and more powerful machines, as machine tools keep growing in size, speed and complexity. 33
The Maker Revolution
Some CNC machine tools are now available in desktop sizes, with prices that bring them within reach of richer western customers. For example, you can now find a desktop CNC milling machine for less than € 5,000, and a desktop laser cutter for about € 12,000. Digital machine tools and non-conventional machine tools also appear in fab labs, makerspaces, and hackerspaces, where they are accessible to members.
The digital maker revolution may be just as unsustainable as the digital fabrication revolution in factories
There is quite some difference between desktop CNC machines and the CNC machines used in industry. A desktop CNC milling machine can only mill wood or aluminium, and a desktop laser cutter only cuts thin wood, plastics or cardboard. However, some fab labs and makerspaces have been introducing industry-scale machine tools lately, and the general expectation is that the possibilities of desktop manufacturing tools will improve quickly. Furthermore, there isn’t a strict separation between the digital revolution inside and outside factories. Consumers can already send digital designs to “cloud manufacturers”, which use industrial-scale digital machine tools to produce whatever you can design on your computer. 34
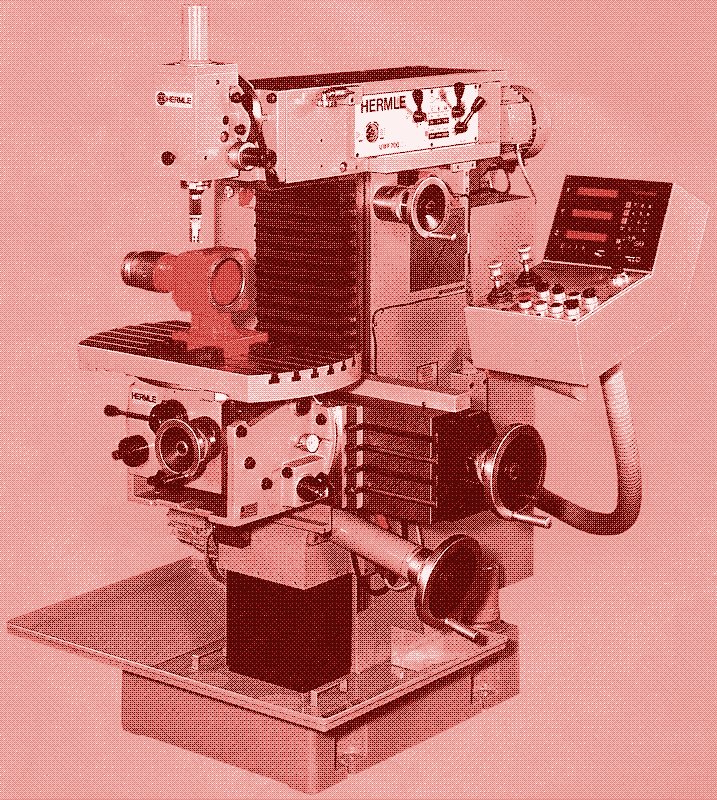
Some environmentalists have embraced digital machine tools. However, this consumer-driven digital maker revolution is just as unsustainable as the digital fabrication revolution in large factories. We’ve been able to produce everything locally for decades using hand-controlled machine tools. Digital tools only allow us to produce more and faster. And this happens, just as in industry, at the expense of higher energy consumption.
It’s not only about the higher energy use of the machines. If consumers can use fast manufacturing machines, total material production will likely increase, similar to what is happening in factories. In his 2012 book Makers: The New Industrial Revolution, Chris Anderson writes that:
“The digital transformation of making stuff is doing more than simply making existing manufacturing more efficient. It’s also extending manufacturing to a hugely expanded population of producers — the existing manufactures plus a lot of regular folk who are becoming entrepreneurs. We will all just be a click away from getting factories to work for us. What do you want to make today?” 34
When billions of people are just a click away from getting factories to work for them, whether in the cloud or on their desktops, this does not bode well for sustainability. We’ll create even more stuff, and each product will cost much more energy than if produced with conventional methods.
«««< Updated upstream
Stashed changes
Reactions
To make a comment, please send an e-mail to solar (at) lowtechmagazine (dot) com. Your e-mail address is not used for other purposes, and will be deleted after the comment is published. If you don’t want your real name to be published, sign the e-mail with the name you want to appear.
Reactions
Matt
Energy use should be normalized against usable parts rendered. Is there any analysis of finished part rejection rates for CNC versus manual methods?
You point out that 7 times more energy is used to produce steel than to machine it. To what extent does using CNC tools allow you to reduce raw material usage? This might be achieved with more complex part designs that are difficult for a human operator to produce, but trivial to a computer reading a 3-D model.
If control and maintenance overhead is a major factor in the energy use of CNC tools shouldn’t you include the energy cost for the care and feeding of 3 shifts of human operators for manually-operated machine tools? I know that sounds a bit crass, but in commercial operations that cost is a huge consideration.
Minor Heretic
Matt has a good point: material use efficiency.
This reminds me of a service provided by the operator of a CNC plasma cutter. They had a program that would take a set of part designs and optimize the layout on a rectangular sheet of metal. Minimum cutting waste. At 10:1 material to milling embodied energy it wouldn’t take much in the way of savings to tip the scale.
Part of the problem is that we don’t pay the true cost of energy, especially electricity. The health costs of power plant emissions, for example, are 1.4 to 3.4 times the retail cost of electricity in the U.S. In some states downwind of a lot of coal plants the health cost is more like 10 times retail. I can’t even imagine what China pays (or fails to pay) for the health costs of industrialization.Including the externalized costs would adjust manufacturer attitudes towards efficiency.
Kris De Decker
Roland (#24),
You write: “Your point carries the implicit assumption that the power of the rest of the machine should go in lock-step with the spindle power.”
In my comment (#19) I write that this is not the case:
“The researchers who composed the table wanted to show the consequences of different levels of automation. And then you see that the difference in total power use is much larger than the difference in spindle power. For example, while the spindle power of the most automated machine is only 11 times higher than that of the manual machine, total power use is 67 times higher. The trend is towards more automation (regardless of the spindle power) and that is what makes digital manufacturing so power hungry.”
One thing remains unclear to me, maybe you could help. You say it’s the working size of the machine that determines spindle power, but the source I refer to in the article says it’s the working speed of the machine:
“Higher production rates require stiff mechanical systems with the capability to absorb arising inertia forces. As a consequence, the masses of the machine structure, such as moving machine components, have to be increased. This, in turn, require motors with high torque output which are able to increase the forces needed during acceleration and deceleration.” [16]
Floris Van Cauwelaert
Thanks for the amazing article. Have to let that marinate a while. Quick question: what if, with renewable energy, we are able take the energy consumption out of the equation? I still feel there could be real opportunity for repair, remanufacturing, creating goods with higher value etc. Secondly, the technology becomes available and cheaper fast, environmentalist have a task of helping to find the most useful applications (and preventing a new wave of gadget-culture). Kind regards, Floris
Mark
This rings close to home, as my tiny company builds an open hardware book scanner using CNC techniques. See http://diybookscanner.eu .
Environmentally speaking, I’m not sure we’re doing as well as I intend. Producing by CNC in a faraway corner of the EU. This is low power wood CNC work, but still. Shipping directly to individual customers all over the EU. I’m flying to Latvia regularly myself (trains take 44 hours and go through Belarus -> transit visa). We do try to minimise plastic packaging.
Do you happen to know about organisations that offer broad eco-analysis for small companies on a budget? I’m thinking production, shipping, product lifecycle, …
ShaneHughes
Great article but only looking at the onsite part of the energy lifecycle is a bit narrow. couple of the more value driven objectives with these fabrication tools is 1. the decentralisation of production which is currently dependent upon shipping materials all across the globe and then parts from different places etc. and 2. production in response to demand rather than our current model which is production as a route to creating demand and which often creates unnecessary surpluses. 3. there are lots of other non energy related stuff like shifting control of production out of the hands of corporations and into the hands of people etc etc..
Better life cycle analysis of full material/energy inputs/outputs are needed and scenario testing etc. its good to be asking these questions though.
I have to say that even if you did a full life cycle analysis i still think that localised digital production would be more carbon/energy intensive than most mass produced long supply chains but the analysis would help understand when its most appropriate.
Mr. Downer
This is a FANTASTIC article, and we need more of this sort of analysis as these new tools come on line, because once they are adopted en masse it will be too late.
In my line of work (architecture) these tools promise to “liberate” design thru making previously difficult forms (compound curves, etc.) much easier to produce - but no one is asking, at what cost?
Specifically, if embodied energies are multiplied orders of magnitude, with a concurrent rise in demand for novelty for novelty’s sake (see, for example, the ungodly & unnecessary variety of tennis shoes as one glimpse into this phenomenae) things like flat glass, straight rolled steel, and other relatively low-embodied production technologies may come to be seen as inferior by criteria that are wholly uninformed by energy and resource concerns.
I imagine there are realms where laser sintering, for example, can actually result in a net energy savings, but we as designers should remain cognizant of the demands so thoughtfully described in this piece, and be careful what we wish for in terms of “freedom”: a world depleted of resources so that humans can “play with form” will be a truly pathetic end, and about as damning of indictment possible for the relatively small cadre entrusted with the creation and maintenance of the built environment.
Andrew Langford
Interesting - I am a small scale shoemaker making mostly standard sizes and fittings but about one third of production is made-to-measure(m-t-m). It is these that I am trying to bring down in cost as, it is not only rich people who have feet that don’t fit standards (!).
Building the lasts (the formers over which boots and shoes are made) is one challenge - starting with a standard last I add patches of leather held down with masking tape. This may take upwards of an hour per foot(these patches get stripped off the last at the finish and, whilst they can be saved for future use, they are not easy to re-attach and get a good result.
Then comes the pattern cutting - again, working out from standard patterns helps but I can’t use the standard pastry-cutter press knives and my cutting press to cut the leather - this has to be done by hand. Making patterns and hand cutting adds another couple of hours to the process.
My standard soles don’t fit, of course, so I either use oversize units and throw away considerable waste or hand-cut again - this is tough to do and not good for hands and arms.
I had been thinking to 3D print lasts, use photo imaging to get to the patterns and then use CAD controlled laser cutting for leather and sole patterns (or, maybe, 3D print soles so as to allow me to make ‘bumper’ soles like those you’d see on many walking sandals - Merril, for example - these days).
I’d not expect this to be a solo operation but one owned by a connected network of shoemakers.
As far as your energy analysis is concerned I’d imagine that, whilst the ‘machining’ might be super energy intensive the whole life picture (locally made shoes that fit, can be repaired, are comfortable and not a mega price - you may not know that 30% of folk don’t wear out their shoes but break them by spliting out the sides because the shoes don’t fit - shoes that fit last 8 to 10 years a pair with resoling every 2 years) still looks better regards ecology than importing Chinese made (child labor?) throw-away jobbies ….
Would love to do the analysis in detail but time is pressing …
G
Mmm however you have to grow and bring the food and water to the person, which may add quite a bit.
Roland Smith
@Mario (#31)
There are a couple of reasons old machines last a long time.
As macona mentions, manual machines are generally used in supporting jobs, not series
production. So they see relatively little use and don’t wear much.
A second reason is that back in the day, machine frames were generally made from cast iron using sand molds because that was the only viable way to make such complicated pieces in series. Since cast iron has limitations on wall thickness (wall thickness generally needs to be >5 mm for cast iron) these frames were generally way heavier and more sturdy than they needed to be.
A reason why modern CNC machines don’t last that long is that computer technology is still evolving. We have a 3D computer controlled measuring machine built in the 1980s. It’s original computer was a box about 50 cm cubed stacked full of custom made circuit boards. When that computer broke down a couple of years ago, the components needed to repair it had been out of production for over a decade. So that computer was replaced by a standard laptop running a much more capable piece of software. On this measuring machine this was a good option. For techical reasons this is generally not a viable option for a CNC milling machine.
Amos Blanton
“A fully automated machine will always consume more energy than a semi-automated machine. So, choosing fewer automated manufacturing technologies should be at least part of the solution.”
I think this statement is an oversimplification. It may be true that the difference in energy costs between the two methodologies is high now, but much of that difference may be a result of our current culture of extravagance towards energy usage. A Raspberry PI computer, at approx. 2 watts under load, should be more than capable of handling the computational requirements of most CNC machines (They just aren’t doing very computationally intensive work.) So if the energy costs at idle are high, it’s most likely down to manufacturers not seeing a clear cost / benefit advantage to minimizing idle power consumption.
I suspect that if most of these machines were designed for an environment in which energy cost was a larger factor of total cost, they would be much more efficient - at least at idle.
Jeremy Faludi
This’s an impressively-researched article. However, I have to argue they came to the wrong conclusion in a couple places:
First, I’ve actually read the Dahmus & Gutowski paper they cited on CNC mill energy use, and the conclusion they came to is not the conclusion Dahmus & Gutowski came to. Their main conclusion was that even the biggest CNC milling energy use was much smaller than the embodied energy of the materials being cut. They say, “the embodied energy per cubic centimeter of input material is around 590 kJ/cm3, or 40 to 120 times larger than the material removal energies” for aluminum, and around 25x for steel. …So at that point, who cares what the CNC machine is doing?
Second, the author’s claim that “Automated machine tools can never become as energy efficient as their hand-controlled counterparts” is just not true. By his own quote of their data, “the human controlled machine requires 6.2 kJ of energy to remove one cubic centimetre of material, while the CNC milling machines require 4.8 to 7.1 kJ of energy”. (And the manual operation number’s not counting the environmental impacts of a person commuting to work, requiring extra factory floor space, etc.) So CNC is sometimes better, sometimes worse.
Third, he says increasing productivity won’t help environmental impacts, “power use increases as the material removal rate does.” But he’s forgetting his earlier quote that 85% of the power use was the same whether the machines are running or not—therefore making more parts in the same time will lower eco-impacts per part, even if you double the 15% of machine power used by the actual cutting. He worries about idle time, but then quotes “these types of machines are often operated 24 hours per day and are idling less than 10% of their total life time.”
Fourth (or, second part of third), he argues “an increase of the production rate also implies an increase in material use,” which is not necessarily so—faster production can just as easily mean fewer tools producing the same number of parts. Smaller factories, and more shared factories (“contract manufacturers”). It, in fact, encourages manufacturing to use the Zipcar business model—the product service system.
A more accurate conclusion for the CNC part of the article would be, “it’s not what you do, it’s the way that you do it.”
However, his conclusions about laser cutting vs. stamping look pretty sound. And he’s right that because energy is cheap, people don’t work to conserve it very much. It’s a low priority compared to cost & quality. He’s also right that when it becomes easier / cheaper to make anything, people make more of it.
Chris Hall
Interesting post Kris, and I’ve also found the follow up comments informative.
I also thought the chart comparing energy use between machines was apples and oranges. A CNC machining center with 22kW at the spindle is a very different animal than the other ones to which it was compared.
The elephant in the room though, alluded to in places in this article, revolves around social factors. As David F. Noble described in considerable detail in his work “Forces of Production: A Social History of Industrial Automation”, the move away from shop floor control of machine tool production, now to the point of ’lights-out’ manufacturing, was quite deliberate. Management very much wanted that control. At every juncture of machine development where there was a choice between a CNC system that required more shop floor operator input, and one that required less, the choice was invariably towards the system that required less shop floor operator oversight.
You cannot reduce the issue of machine development simply to an equation regarding relative efficiencies of energy usage. Less energy intensiveness is a definite plus, however it does not hold sway in the question over what technologies move forward and which do not.
Even if we’re all in agreement that skilled work (operating a manual milling machine or lathe, say) is better for people than unskilled work (i.e., loading materials in and out of CNC machining centers), that manually-operated machines have some advantages over CNC-based ones in terms of energy intensiveness, at the end of the day it is social factors (who controls production?) that have bigger sway.
And if we reverted to a more human-intensive development of skilled operators and lower energy machining practices, where would these operators come from? Our educational system is not producing them. Every young person is supposed to go to college and get a job in the financial sector or other white collar pursuit.
Jason Olshefsky
I was appreciative of the attention to detail in this article, but was resistant to any negative view of CNC as I frequently call upon shops to use CNC to build parts for my company. While I don’t think CNC is wholesale condemned, I think it gets undeserved attention: like several other commentators, I think it’s the social behavior that is at fault, not the tool. An analogy would be to blame the hammer since one person can injure another—it’s not the tool, it’s the operator. For CNC, it’s the culture of creating artificial demand, planned obsolescence, demanding cheapness, and other socially-egregious practices.
Nonetheless, it is another factor for me to consider when deciding how to get things manufactured. As it stands now, I think I am firmly on socially-conscious ground in requesting parts only when needed (reducing wasteful production), using recyclable materials (for end-of-life either through wear or damage), and attempting to find shops that implement good labor practices (fair pay, safe environment, and unionization where needed). I’ll add to that considerations of energy efficiency: for parts that could be made on manual machines or by CNC, I’ll favor manual machining.
Ryan
What about additive manufacturing like 3D printing? -I read an article that showed even though it is highly automated it uses less energy and has less emissions than conventional manufacturing. See https://www.academia.edu/4685670/Environmental_Life_Cycle_Analysis_of_Distributed_3-D_Printing_and_Conventional_Manufacturing_of_Polymer_Products
John Fisher
Someone may have mentioned this, if so sorry, but the units used in the chart of comparative energy use are incorrect. the correct unit is Kwh kilowatt hours, not Kw.
For instance a 6700W spindle head in a CNC center may only move for a few seconds, as the processes are much much faster than a manual machines. So -just making up numbers - a 1 second CNC cut at 5000W is 5000 W/seconds or .0013 Kwh where a 20 second manual cut on a spindle at 1000W is .005 Kwh.
Also the CNC works much much faster than a manual system, so you have to figure out the energy per part cost.
That said, its perfectly true that there are motors and computers in the CNC that don’t even exist in the manual machine, where some movements are powered by breakfast and lunch. However fewer employees also means fewer car trips and less energy overhead.
Life-cycle costs are a big headache!
macona
This article is terrible. So many things wrong. I work as a tech in one of the largest job machine shops on the west coast.
As one other has said, just because you have a spindle rated at 108hp (like we do) does not mean it draws anywhere near that much power. I dont think we have ever had that spindle close to that point. It only draws as much power as the load requires.
This applied to lasers too, one of our lasers is 4.4kw but is rarely ran at that power. Material type and thickness determines power setting. Along with our two lasers we have two cnc punches. They are crazy fast and kind of fun to watch but they also require a lot of maintenance and tooling is rather expensive. Plus you really need a tool shop to keep the tools sharp. Then there is setup, a laser you can just send the file down that is generated from a cad file, you can also program at the station with it’s build in cad software. Enter the parameters of the material and how many you want and go. The punch is a different story. You need special tooling for anything but the most basic of designs. And you need someone to set the machine up. The punch does do things that the laser cant do, like raised tabs, louvers, and countersunk holes.
“We’ve been able to produce everything locally for decades using hand-controlled machine tools. Digital tools only allow us to produce more and faster. "
Nom just no. Before we had cnc lathes we had screw machines, tracer lathes, and turret lathes. These are what we used for production of any decent quantity of parts. They take time to set up but once you do it is basically pull a lever and go. The screw machines will run unattended as long as you have stock feeding it. “Lights out” operations existed before “Digital”.
Before cnc mills we had tracer mills. These are single or multi spindle machines that used hydraulic or air powered tracers to copy 2 and 3d parts from a master making multiple parts at a time. They even had these set up with 4th axis attachments to do very complex shapes like turbine wheels.
Production has almost never been on traditional manual mills and lathes. Manual machines in production shops were mostly found to support or repair the production machines and build and maintain tools and dies. Manual machines were and are still found in small shops like oil field shops and home shops too. Even there they are being replaced with cnc.
Many people thing CNC is only good for production, it is also good for one offs. Many machine have what is called “conversational” where you can design and run a part right at the machine. You can also enter manual commands from the MDI.
Manual machined parts mean a lot more scrapped parts from things like manual goofs or just not being in tolerance. You probably loose more money here than any place else, especially on a multi op piece.
The author seems to think you can just take a design and pop it out with no one to attend to it. It does not work like that. Sorry. Most parts are multi op parts. Stock is prepared, fixtures are designed and built, multiple machines are used, debur, finishing, etc. That is why this “manufacturing a click away” will never happen. You can easily design a fancy part, that does not mean it can be made. It takes programmers to program the part and select tooling and to figure out how the heck they are going to make it. Then you need to figure out how you are going to hold the part down, this often requires fixturing.
The author really need to spend some time in a modern machine shop to see how things work before he writes an article trying to trash it.
Mario Stoltz
One question that really interests me as a general takeaway.
my gut feeling would be that in pre-industrial production (by smiths and craftspeople), most energy in the system was embodied energy of the materials. I would think that the energy embodied in the manufacturing tools and their operation was small compared to the embodied energy of the materials. This is as most tools did not have a lot of wear. Those tools that did easily wear or break were small and easily replaced.
With industrialization, the ratio between “tool energy” and “material energy” started to change. Embodied energy of the tools (real machines for the first time in history = larger, heavier, much more complex) and the engery for their operation (steam power) was fairly high. Of course, productivity also rose steeply. Still (again - gut feeling only) I would assume that the tools were mostly built to last and thus the embodied energy of the materials was still higher than that of the tools and their operation. This is indicated by the fact that many such old machines have worked for 50+ years and some are still operational even today.
With modern manufacturing, I would not be sure of the result. Embodied energy of tools (like 5-axis CNC machining centers) and energy for their operation is considerable. The lifetime of such a manufacturing station is maybe 10 or 20 years, or less? I cannot imagine that they any of those operational today are still around in 2050. For the production of non-metal components, we predominantly use power tools rather than manual tools. Also these have a shorter lifetime and higher embodied energy than any manual tools. On the other hand, their productivity is also higher.
At the same time, the materials that we use in construction nearly all have higher embodied energy than classical materials. We use light metal alloys or plastic composites rather than wood. Nearly none of the semi-finished materials we typically use can be provided without a factory that processes them in large machines; this is true even for the wood-based materials (plywood, chipboard, MDF).
Two questions remain:
how has the ratio of manufacturing energy to material (embodied) energy developed, and especially for modern-day production, is it really higher than the historical average or not - taking into account the productivity increase?
how are we looking in terms of the general embodied energy in the things that we use and in which we live and work today? Here, the answer is clear. Embodied energy in anything that humans manufacture in the first and second world has exploded and is exponentially higher than the embodied energy of the things that our ancestors have used. This alone is enough to kill humanity if we do not change it. This is where our real problem is.
kris de decker
@ Mario,
I have numbers that confirm your gut feeling.
In general, energy consumption during the use phase dominates total machine tool energy use, reflecting both the high energy use during the use phase and the long life expectancy of machine tools. However, while the average lifetime expectancy of human-controlled machine tools amounts to 18.6 years, the average lifetime of a CNC machine tool is only 9.5 years.
Source: [12], page 26 and further. http://www.ecomachinetools.eu/typo/reports.html?file=tl_files/pdf/EuP_Lot5_Task2_August2012_FINAL.pdf
The numbers concern metal working machine tools in general. The document also has figures for other types of machine tools and for individual types of metal working machine tools.
Voxus
I’d be curious to know how human energy is factored into the equation. The humans who would be controlling the more efficient machine aren’t directly using electricity to function (as a computer controlled machine does) but they consume a ton of other resources indirectly. For example, the humans eat food, drove to the factory, heat their house, fly around the world — all of which consumes energy so you can’t just ignore the human energy variable as it is quite significant over a lifetime.
Tanjil
CNC machines are much better than manually operated machines. I know the power consumption for running a CNC machine is much higher but if you look at the time saved by these machines then we can’t think of any alternatives. Sometime it is not feasible for small manufacturing companies to afford this high priced machines. For them , manually operated Lathe, milling, drilling, shaper or planer machines are a good choice. And if we talk about the longevity of these CNC machines, then we have to think out of the box. Mechanical, Chemical, Metallurgical engineers have to work together to make a prototype which lasts longer and also less expensive like manually operated machines. This will certainly help the economy. But again if we think about the time saved and quantity of the products that a computer operated machine produces we can’t complaint ! And again the power consumption! (off topic) Engineers and scientists please suggest the world to use non-conventional energy sources or renewable energy sources. Thanks
jon banquer
Just came across this site and in general, enjoy your articles. Most of them seem well thought out and documented. However, this one …
The description of how nc operates is very good, especially for a person who is not in that field. But your conclusions …. mmm, bad boy :)
I don’t disagree with you on the social end of things : if I were to start a shop now it would be all manual. But the reasons are not energy-related.
Bona fides : I started and ran a small shop from about 1976 until a couple years ago, so I’ve actually done production on manual machines. There aren’t so many people around now who can make that claim. I also
bought and paid for and did all the accounting for when I went to nc. I’m more of a lathe guy than a miller but the principles are the same … let me throw out a few things for you to think about :
When I got my first cnc lathe in 1978, I ran the same jobs on it that I’d been doing manually. I’m a decent lathe hand, by the way, so it’s not like we’re comparing some slouch to a hot-dog cnc machine.
The nc machine was almost exactly ten times faster. So for the exact same quantity of parts you would need ten lathes and ten guys, all eating lunch, pooping, driving to work, parking out front. Ten times the footprint. This is not energy-efficient !
Engine lathe, 6,500 lbs x 10 = 65,000 lbs. One nc lathe, 20,000 lbs (This was in the old days, a new one that size generally runs maybe 12,000 lbs) Energy to produce ?
NC produces way higher quality. The tolerances were better and there were shapes that could only be done with great difficulty (if at all) by hand. Many features simply can’t be done by hand. Parts off an nc machine are several orders of magnitude more accurate.
Scrap : on the nc machine I would often scrap the first part because it was easier to just make one, measure it, then change the offsets. Afterwards, unless a tool broke, there was no scrap. In the old days we figured 4% scrap. So if you needed 100 parts, you’d cut up 105 - the last thing you want is to come up one part short on an order so you’d figure the 4%, then add one or two.
Where’d that energy go ? :)
@ Mario & Roland Smith : another reason manual machines last longer is that you simply can’t run them hard enough to hurt them. Okay, you can hurt them but it’s difficult for the human body to withstand the
torrent of blue-hot chips flying off a machine that’s
really working. The reason I bought my first nc was because I was sick and tired of chip burns all over my body. Arms, down your shirt, in your ears. You can’t enclose a manual machine because you have to get
to the levers. There’s another point against manual
machines : the mess everywhere. How much energy does it take to clean up all the chips, and how bad for the world is all the coolant that gets sprayed everywhere uncontained ? This may seem inconsequential
but I’d have to clean out the chip pans at least once a day, often more. NC machines almost all have chip conveyors that (sort-of) dry the chips and dispose of them into barrels. And the oily ones have Roto-Mist oil collectors that filter the coolant out of the enclosure. Without an enclosure you can’t do that as very well.Clean the air for the entire shop … does that take energy ? hint hint ?
I sort of wonder if the people who studied manual machines actually had any manual machines to study : it’s been a long time since there were any manual shops. Anything bigger than a Bridgeport is always idling. You don’t turn the motors on and off. A typical 16" engine lathe will have a 7.5 horspower AC motor that gets turned
on, then it runs. All the functions are controlled by levers and clutches. Any milling machine bigger than a toolroom mill is the same. Manuals idle more than nc. Manuals are always idling.
Think of ten 7.5 hp motors running continuously versus one 20 hp plus the two servos (one for each axis), for the same amount of product. I don’t think the manual will come out more efficient.
Worse, a lot of production equipment was powered by hydraulics. All the tracer machines, both mill and lathe, ran hydraulic tracers. Let me tell ya, hydraulic systems are not energy efficient ! Nor are they clean. Many larger machines have hydraulics to do the actual work. Not energy-efficient and they are always idling at full energy draw, whether they are producing parts or not. The pump is producing 250 psi all the time, not just when doing work.
Another statement that sticks in my craw is the claim that nc machines while idling eat up a lot of energy. I simply don’t buy that. You said, " … the power used handling the workpiece and tools is less than 15% of the total power required. The remaining 85% of the power used by the machine is constant, even when no action takes place." But the point you are missing is, even if this were true, if a company doesn’t want to go broke the idle time is infinitesimal. You open the door, take out the part with an air chuck and button push, put in another part, close the door, push “go”. Many machines now change parts, too - in just a few seconds. So, for smaller parts, maybe
fifteen seconds every five minutes of machining time. On large parts, the machine can run for hours between part changes. The key to making money is “Keep them spindles turning !” and that’s what shops
do.
Remember, nc machines are expensive. If a shop has its nc idling a lot, then they will quickly go broke and someone will buy those machines at auction who does not leave them idling unproductively :)
Even when idling, I don’t buy the claim that they are inefficient. Of course there are always improvements to be made but a 1980 cnc machine was much less efficient than a new one, and the 1980 machine was even
then way more energy efficient than a manual. My electric bills about tripled but I got ten times the volume. Plus back then the electronics required an air conditioner, the transformers gobbled electricity, the motors and servos were all dc. I have no numbers to throw out but I
know that a 2012 machine uses way less electricity, even sitting there, than a 1980 machine. I just can’t believe this thing about nc not being ’energy efficient’. It goes 180* counter to my real-world experience.
If you remember, in the seventies we had two oil embargoes which jacked up the price of electricity by two and three times. Many of the “authorities” predicted big price increases because of that, but the
machine builders and utilities replaced all their older motors with more efficient ones instead, and we didn’t see what the ivory tower ’experts’ predicted. The manufacturers and users are not as unconcerned with electricity prices as you think.
Anyway, at least on all my machines, when the spindle is not turning the spindle motor is off. Contactor dropped out. No current going through it. That’s much more efficient than a 7.5 hp motor continuously turning, yes ? The ballscrew motors as well : if there is a turning force on the screws, then the current delivered to the motors goes up. But with no force, current to the motors is infinitesimal. When they are moving, they eat a lot of electricity - but that’s because they move at 400 inches per minute. Try that with a manual machine :)
Another point - nc machines use ballscrews. Manual machines use Acme-thread lead screws. There’s a tremendous frictional difference there. Yes, the cnc most likely does use more total energy - but that’s because it is moving ten times faser and making ten times as many parts per unit of time.
This is possibly where your analysis went off the rails : what you really need to know is the energy used per part. If you did that I am sure that NC machines would clean the floor with manuals.
You bring up lasers. Yes, lasers are energy-intensive. They are also maintenance-intensive and cost a lot. No one who pays the bills likes them because honestly they cost too much and you are right, they eat energy. Pieces of junk, really … But they are so versatile ! (and clean. Punches are not clean, all that stock has to be oiled to be
punched. No one mentioned that to you, right ? Then the oil cleaned off it. Lasers don’t need that.) So, let’s assume that you have a shop where half the work could be done by punching, but the rest needs a laser. Why would you buy two machines ? Yes, the laser is too expensive and too much hassle to be optimal for the job that’s
suitable for punching and it drinks electricity (aka energy) - but you don’t have to buy a second machine ! These machines are not small, either. To handle a 4 x 8 sheet of steel the machine has to be at least twice as big as that, yes ? (The steel moves, not the cutting
head.)
So how much energy do you save by not having that second machine, floor space, oil, and operator ? Probably more in one week than the laser will waste by being a high-energy process in its entire lifetime
…
Don’t get me started on “3d printing” – what a fraud :)
I’m sure I’ll think of more holes in your article :) but that’s enough for now. I do agree with you about the social side of it - I’d prefer that nc machines were never invented. The throwaway economy filled with cheap junk is anathema to me. But really, nc machines are not less energy-efficient than humans. You are mistaken on this one.
========
Anyhoo, except for this one article, I like your magazine. Some good thinking and analyses there.
best of luck !
Jon B
JohnMc
The line – “Because energy consumption equals power consumption multiplied by time, in the end a CNC machine might use less energy than a manual milling machine for the processing of a similar part.” Pretty much punches a hole in the balance of the article. For what really matters is not how much overall energy is utilized but the imputed Kw per part produced. I would suggest that industry has already figured this out, else-wise they would be at a competitive disadvantage vs their competitors for the energy cost of every part made.
Randy Eckart
@Kris, when you get around to that 3D printing article, you might look into Direct Shell Production Casting technology.
http://engineershandbook.com/RapidPrototyping/dspcrt.htm
With this method the ceramic molds for metal castings are printed, and then fired and poured with molten metal.
Shapeways has a similar but more direct process where a 420 stainless steel metal powder is 3D printed with binder, and then infused with bronze in an oven for a final composition of 60% steel / 40% bronze.
http://www.shapeways.com/materials/steel
Compared to direct laser sintering of metal powder, either method should be much more energy efficient. In fact these methods should compare quite well to traditional metal casting technology in terms of energy consumption.
AndydV
@Kris et Al, A very interesting article and discussion. For anyone who wants to assess eco-efficiency or attempt LCA (life cycle analysis) there are some wonderful free resources at Tech University Delft. Excel spreadsheets of Reputable Idemat data can be down-loaded giving individual eco-indicators (carbon eq, energy intensity etc) and compounded eco-cost data for thousands of materials and processes. http://www.ecocostsvalue.com/EVR/model/theory/5-data.html
The need and approach for LCA are explained elsewhere on the site (click on home page at the top R). A common approach is to calculate the burden per kg of product from its constituent components (materials & processes). The boundary of the analysis can be selected to suit the application from comparison of competing processes to cradle-to-grave life cycle analysis taking account of packing,transport,usage,recycling, disposal etc.
Be very careful when comparing process data ….eg material processed in injection moulding is the mass of part …for milling it is the material removed (which may be half of the blank mass)…but for sawing,laser or water-jet cutting it is the material removed in the kerf (not material cut away)….you have to keep your brain engaged! Some very quick assessments based on Energy intensity or eco-cost can indicate where the major eco-burdens lie..Find the big ones..assess which are addressable..then go for the low-hanging fruit!
Guðni
Nothing about a abrasive waterjet, they can even cut a 3D material with great precision and with minimum of a heat deflecting on the material. Please add an article on your take on the matter